Chapter 5 ~ Biodegradable and Non-biodegradable Waste
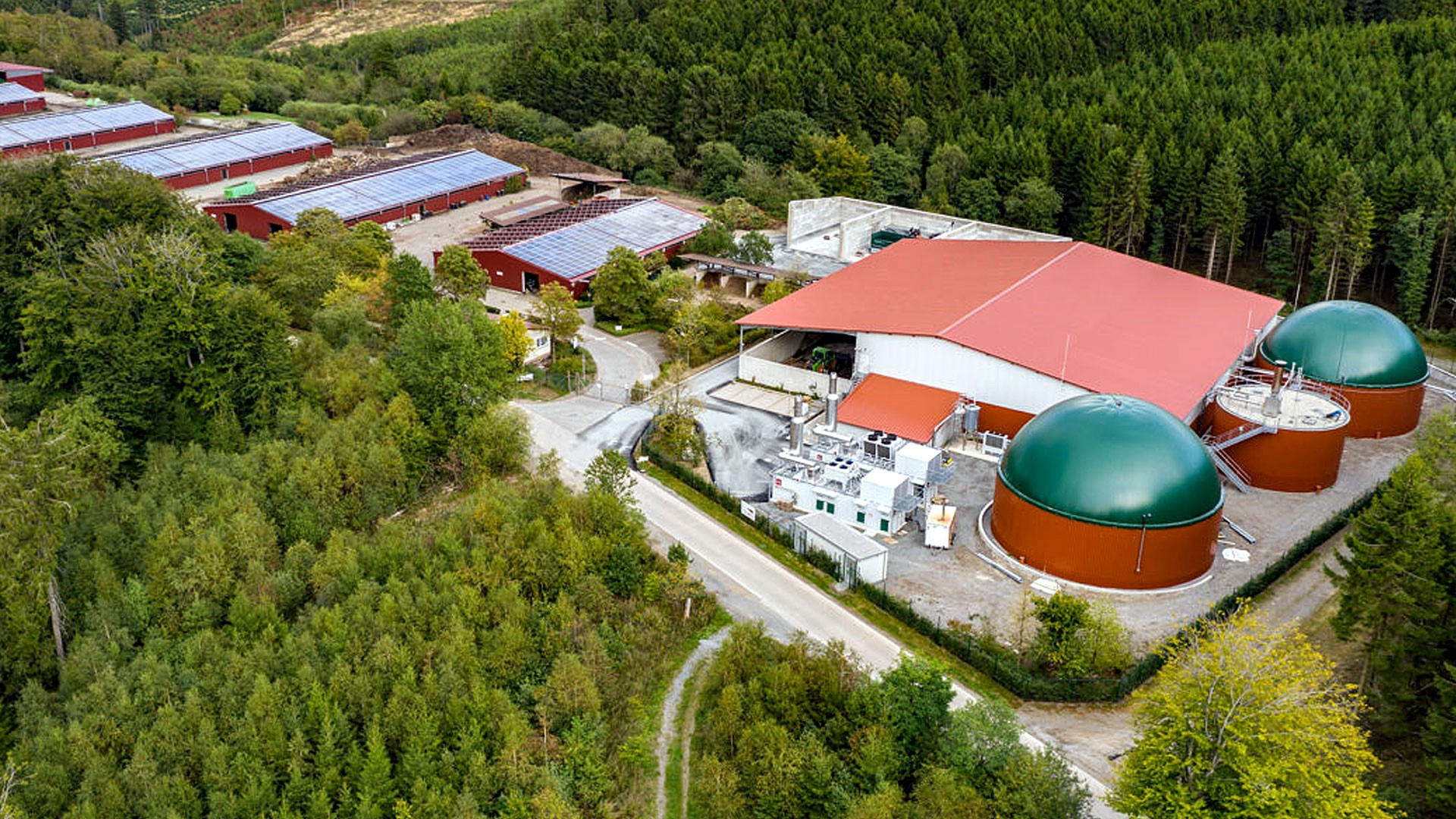
Key Terms
Hazardous, e-waste, bioaerosol, heavy metals, pesticides, fertilizer, nanoplastic, radioactive waste
Learning Objectives
Upon completion of this chapter, students will be able to:
- Describe a way that biodegradable and non-biodegradable substances are constantly being added to the environment.
- Explain types of biodegradable waste produced by humans from agriculture and non-agricultural sources.
- Differentiate among the non-biodegradable substances that are increasing in our environment.
- Detail the impact of plastic pollution as nanoplastics are creating a different level of pollutants in our environment.
- Identify environmental pollutants in terms of organic and inorganic substances and cumbersome metal generation.
- Explain how municipality waste is becoming an environmental hazard.
- Identify waste management strategies taken by the government and non-profit organizations.
Chapter Overview
- Introduction
- Classification of Waste
- Global Generation of Waste
- Bioaerosol from Biodegradable Waste
- Plastic Waste
- Heavy Metal Generation
- Problems Caused by Biodegradable and Non-biodegradable Waste
- Conclusion
Introduction
Waste from various sources is an increasing concern for the environment, as the amount of waste we produce daily is enormous. Biodegradable waste is substances that can be decomposed or degraded by microorganisms and other living organisms. These substances are mainly added to the environment from plant and animal sources. Some biodegradable substances are decomposed easily in the natural environment, and some take a long time to degrade. Non-biodegradable waste is mainly inorganic substances such as plastics, cans, chemicals, bottles, etc. Both biodegradable and non-biodegradable waste have some adverse effects on the environment, as both cause pollution that makes our habitat inhabitable. Billions of tons of waste are generated every year. In this chapter, we are going to discuss the type of biodegradable and non-biodegradable waste produced as industrial waste, commercial waste, agricultural waste, domestic waste, and municipal waste and the short-term and long-term consequences of this waste. Also, this chapter looks at various strategies that have been undertaken by international, government, and non-government organizations to reduce and manage waste materials.
Classification of Waste
Waste is a product or substance that is no longer suited for its intended use. Biodegradable and non-biodegradable waste are the two broad categories of waste.
In a natural ecosystem, the decomposable substances and the gaseous substances generated from animal and plant sources (dead organic matter) are utilized as food or reactants. But very often, some of the substances resulting from humans often take a long time to degrade. Therefore, the definition of waste is based on the concept of waste removal or disposal, as we can see across these definitions:
- According to the 2019 Basel Convention, “Wastes are substances or objects which are disposed of or are intended to be disposed of or are required to be disposed of by the provisions of national law” (Article 5 Basel Convention, 2019).
- The Organization for Economic Co-operation and Development (OECD) states, “Wastes are substances or objects, other than radioactive materials covered by other international agreements, which: i) are disposed of or are being recovered; or ii) are intended to be disposed of or recovered; or iii) are required, by the provisions of national law, to be disposed of or recovered” (OECD Report, 2023).
Around the globe, the definition of waste varies, and different approaches are used to classify the waste material. Recycling and reusable materials are often classified as waste, since these are discarded products that can be usable only if certain activities are completed and documented. Alternatively, industrial by-products may not be considered waste under certain conditions because of the value-added products that can be generated.
Waste can be classified based on source (who/what generated the waste?), substance (what is it made of?), hazard properties (how dangerous is it?), management (who handles it?), or a mix of these concepts.
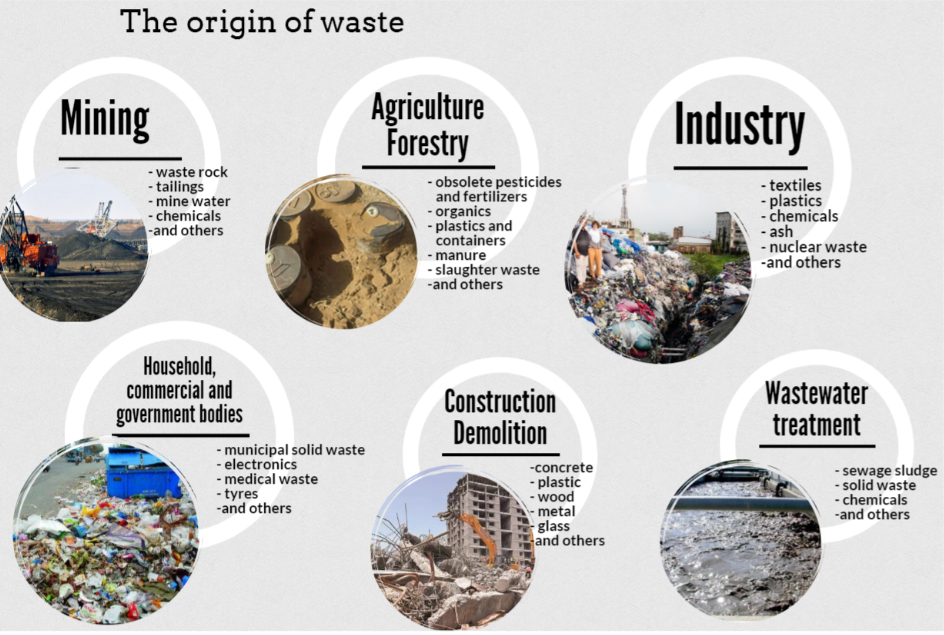
Two main waste categories of waste are typical: non-hazardous or solid waste and hazardous waste:
- Non-hazardous/solid waste materials are not severely harmful to human and/or animal health. This includes plastics, beverage cans, bottles, and organic waste. Still, non-hazardous waste can have a serious impact on health and the environment if it is not properly managed (collected or recycled, for example). A large proportion of solid waste can be recycled and reused if the waste is collected appropriately.
- Hazardous waste has the potential to cause harm to humans, other organisms, or the environment. This waste needs special attention for treatment and handling. Chemical and physical characteristics determine the exact collection and recycling process. Flammability, corrosiveness, toxicity, ecotoxicity, and explosiveness are the main characteristics of hazardous waste. Liquid, gaseous, and powder waste need special treatment by default to avoid the dispersal of the waste. Generally, separate collection and handling are established to avoid contact with non-hazardous waste. Chemical treatment, incineration or high-temperature treatment, safe storage, recovery, and recycling are possible modes of treatment for hazardous waste. Most hazardous waste originates from industrial production. Special kinds of hazardous waste include:
- E-waste is waste from electric and electronic equipment, such as end-of-life computers, phones, and home appliances. E-waste is generally classified as hazardous because it contains toxic components, predominantly lead and mercury.
- Medical waste originates from health care facilities, including hospitals, doctors’ offices, veterinary clinics, and dental practices. This usually consists of medicines, chemicals, pharmaceuticals, and materials contaminated by potentially infectious materials, such as blood or body fluid. Medical waste can be infectious, toxic, or radioactive or contain bacteria and harmful microorganisms (including those that are drug-resistant).
- Radioactive waste contains radioactive materials. This waste can occur as a by-product of industries, including nuclear power generation, uranium mining, medicine, and certain types of scientific research. It is hazardous because it contains or emits radioactive particles, which can be harmful to human health and the environment.
Global Generation of Waste
Data on the global generation of waste is not accurate due to gaps, overlaps, and waste classifications varying from country to country. For per capita waste production, high-income countries produce far more of the world’s waste. World Bank data reports that waste rates will be growing worldwide, and by 2050, it is anticipated that global waste will increase by 70% on 2018 levels (World Bank, 2018). In low-income countries, waste management infrastructure is very poorly developed, resulting in over 90% of the waste being mismanaged. Figure 5.3 illustrates the predicted waste generation by the year 2050.
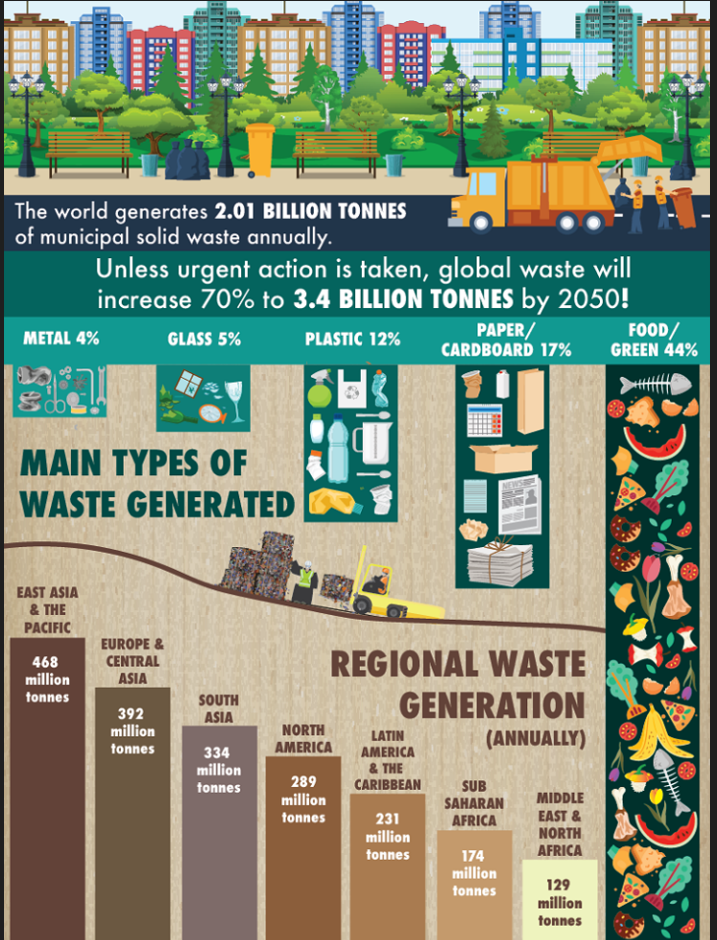
Lower-income countries produce higher amounts of organic waste, whereas higher-income countries produce more non-biodegradable waste, such as paper and inorganic materials.
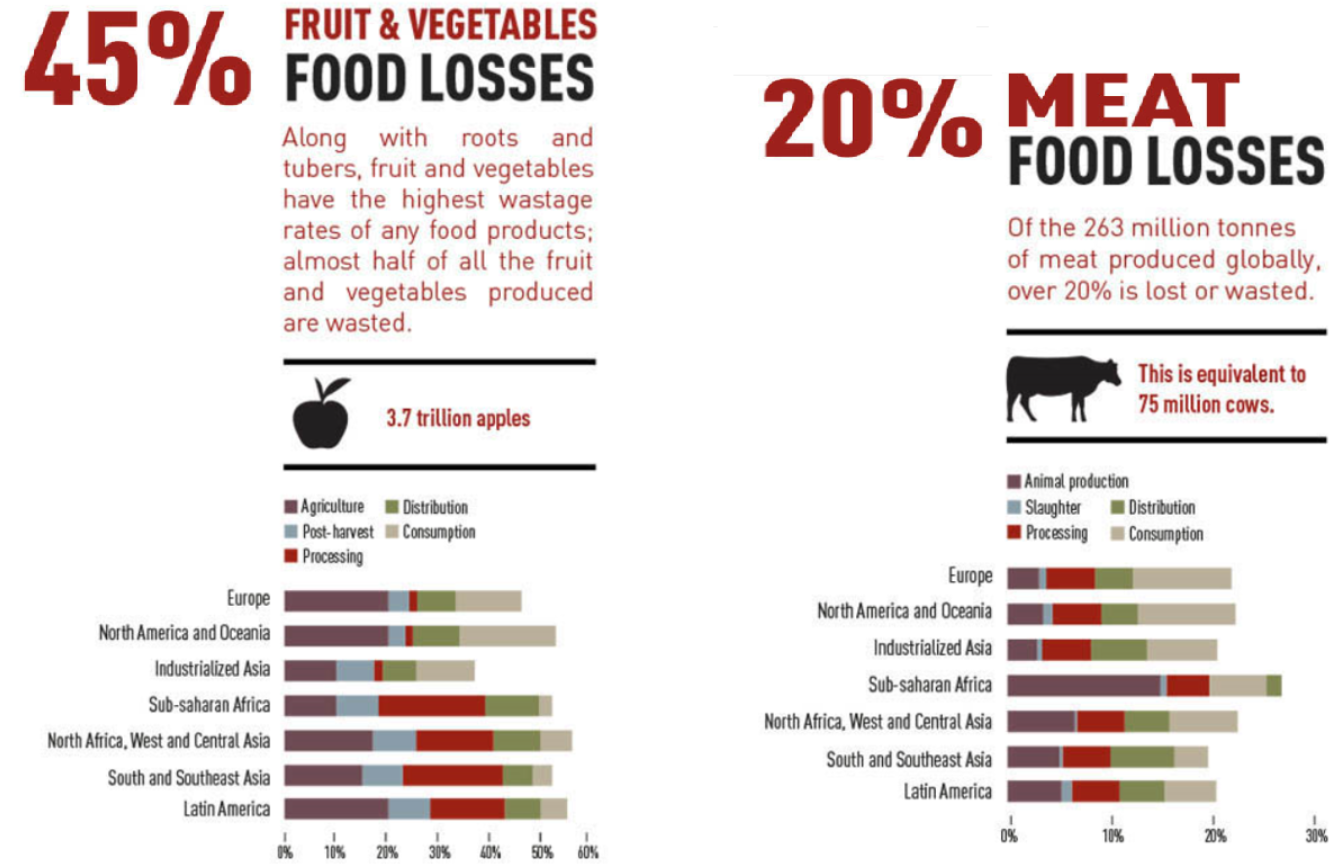
In the case of food waste, roughly one-third of the food produced in the world for human consumption every year—approximately 1.3 billion tons—is lost or wasted (FAO 2019). Food loss and food waste are often separately distinguished, with food loss referring to food produced but not consumed before reaching consumers due to bruising, wilting, etc., in the earlier stages of production and transport, and food waste meaning the discarding of good quality food at the retail or consumer end of the chain. In developing countries, 40% of losses and waste occur at post-harvest and processing levels; in industrialized countries, more than 40% of losses and waste of the whole amount of food produced happen at the retail and consumer levels.
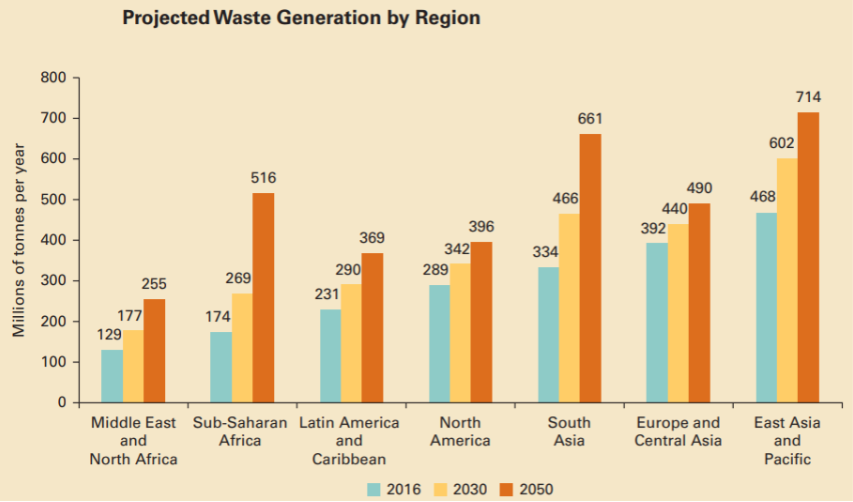
Bioaerosol from Biodegradable Waste
Biodegradable waste generates bioaerosols, and non-biodegradable waste produces heavy metals. These two categories are essential even after following the proper protocol of solid waste management (mechanical or bioremediation).
Microbiological activity during the biodegradation of waste in this section occurs through the activity of metabolism, growth, and reproduction of microbes. The microbial activity is the fundamental mechanism for the conversion of composting, and therefore, management of this waste (blending, mixing, turning, aeration, and screening) produces a substantial amount of aerobic and anaerobic bioaerosols and may generate potential risk to plant workers and the adjacent population (Pearson, 2015). Bioaerosols contain several live or dead microorganisms suspended in the air, such as spores of fungi (Penicillium, Aspergillus), fungal spores, bacterial fragments, endotoxins, mycotoxins, and metabolic end products (glucans and endotoxins). The bioaerosol of compost plants contains a large number of bacteria (8×106) and fungal (2×107cfu) 106 colony-forming units per cubic meter (Pearson, 2015). A study conducted to evaluate biological entities in bioaerosol revealed that the air samples near the shredding area and processing operation unit contain the highest amount of dust, pathogen, endotoxin, and antigen (O’Connor et al. 2015). Another study reported that the mixing of compost generated more toxic (β-1,3 glucan and endotoxin) bioaerosols than the static compost (Sykes et al., 2011).
Bioaerosol Management Strategies
Inside composting, plant bioaerosol is shown to penetrate into the respiratory system due to the minute particle size. Many studies evaluated the effect of aerosol penetration on plant workers. They found that long-term exposure to the compost shredding area can cause respiratory distress, mucosal membrane irritation in the eyes, and impaired lung function among laborers working in the plants (van Kampen et al., 2014). Based on the health effects on workers, a strong approach is undertaken and adopted to reduce occupational exposure to these aerosols. High-efficiency filtration devices are used to reduce aerosol mixing, especially in front-end loading doors in indoor or outdoor composting plants. A high level of protection is taken to clean the filters, clean the vehicle cab, show cleaning, and mitigate leakage in the filter cleaning system. Bioaerosol evaluation outside the compost area is challenging. The bioaerosol near the area of the plant showed A. fumigatus in a large number, but dispersion due to downstream wind reduces the concentration of the microorganism and other endotoxin and metabolite concentration. Due to the limitation of detecting the level of hazardous elements, no absolute consensus can be created. But the findings of these reports contributed greatly to the development of governmental policies.
Plastic Waste
Plastic waste is the most important sector of waste management. The use of plastic and the production of plastic materials have created a critical concern for environmental pollution, especially in the oceans. Figure 5.6 depicts the estimated number of plastics disposed of in seawater.
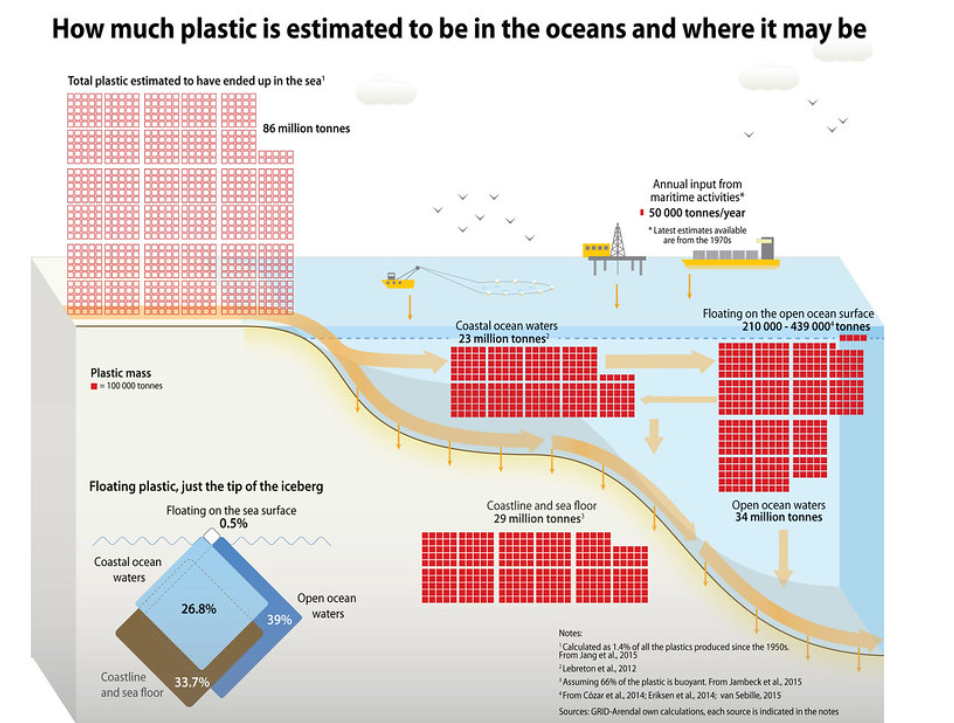
Nanoplastic from Non-biodegradable Plastic Waste
Plastics are made of stable chemicals, and due to this stability and persistence of the chemicals, bioaccumulation of plastic chemicals causes a concerning and prominent situation for the environment. The abundant use of plastics has covered the earth’s surfaces, and plastic was detected in every place such as in soils (Hu et al., 2019), lakes (Xiong et al., 2018b), oceans (Mendosa et al., 2018), and most interestingly, in the places where human activity is mere, such as Antarctica (Munari et al., 2017) and Arctic region (Peekan et al., 2018). Although plastic degradation is prolonged and takes prolonged time, it can be degraded into minute particles due to weathering, radiation from sunlight, and biodegrading entities. The relatively bigger particle sizes but smaller than 5 mm were considered microplastics (Thompson et al., 2004). The other abundant sources of microplastic are cosmetics and detergents (Lei et al., 2017). Due to their smaller size and low density, microplastics can leach the traditional wastewater treatment and flow into the sea (Murphy et al., 2016). These microplastics further degraded to nano-sized plastics (1nm–100nm) (Jambeck et al., 2015). The product formulated with nano-sized plastics is also an important source of nano-sized plastics in the environment.
Effect of Nanoplastics on the Ecosystem
The degradation process of microplastics to nanoplastics is not very well understood, but the degradation process is undoubtedly a continuing process, and the decomposition of microplastics accumulates an enormous number of nanoplastic particles in the respective places where large amounts of microplastics form gyre (Mattsson et al., 2015). Still, no effective quantification method is available to determine the quantity of nanoplastics in the environment. The University of Minnesota has tried to determine the amount of microplastics present in water drinking water bottles (see Figure 5.7; Mason et al., 2018). Eleven brands of drinking bottled water from the US and around the world were tested, and the average number of microplastics across the brand was 325 microplastic particles per liter of bottled water. One sample from the brand contains more than 10,000 microplastic particles per liter of water. Types of microplastics found in bottled water are polypropylene, nylon, polystyrene, polyethylene, polyester, polyethylene terephthalate, azlon, polyacrylates, etc. (Mason et al., 2018). Nanoplastics in the aquatic environment are easily ingested by aquatic organisms because of their particle size and the widely distributed nature. The presence of nanoplastics was found in the body of zebrafish (Lu et al., 2016). The author also reported that the concentration of 20 µg/ liter (1.1 X108) particles can cause localized infection and lipid accumulation in the liver, causing metabolic disorders and disruption of cyclic patterns of energy. The presence of nanoplastics has been reported in the different body parts like viscera, gills, liver, testis, and blood of Oryzias latipes (Kashiwada, 2006). The size and concentration of the polystyrene nanoplastics were 39.4 nm and 10 mg L-1. The presence of nanoplastics in the blood, liver, and other organs indicates that polystyrene nanoparticles can pass through the blood-brain barrier. The blood-brain barrier in the animal system protects the entry of neurotoxins and prevents them from entering an active transport system controlled by p-glycoprotein. This triggers the possibility of polystyrene accumulation in the organism’s central nervous system and might cause severe effects. The accumulation in the body of the organisms allows the transport to the higher trophic level of the food chain, such as fish and birds, and eventually in humans. Even a low concentration of nanoplastics (0.01%) could be transported to the food chain from algae to zooplankton and fish and remarkably affect the metabolism and feeding behavior of the fishes (Cedervall et al., 2012).
Researchers have noted the chemical absorbability of nanoplastics, including heavy metals, polycyclic aromatic hydrocarbons, and polychlorinated hydrocarbons, and the aggravated nature of these chemicals affect living organisms adversely (Koelmans et al., 2016). The ingested chemicals by nanoplastics can form a nanoplastic-contaminant complex, and simultaneous absorption of these chemicals continues. During the degradation process of plastics, many toxic chemicals are released that involve plastic additives, bisphenol A, polymer monomers, polybrominated diphenyl ethers, and phthalates. These chemicals can cause acute poisoning and initiate endocrine disorders and toxicity to the reproductive system (Bejgarn et al. 2015). Based on this evidence, nanoplastic contagion should be taken as serious, and the level of ecotoxicity and the risk of environmental pollution need to be well understood. The toxicity results of nanoplastics are still limited, and further research is still required. Most of the studies were conducted on model organisms like zebrafish and earthworms, and a few toxicity studies were performed in mammals. As the metabolic system in lower-level and higher-level organisms is different, thus the effect of toxicity upon the entry of nanoplastics into the subcellular level and in the blood circulation system will be different in these two levels of organisms when considering the ability of clearance and resistance to the nanoplastic toxicant. Many factors affect the release of toxicants from the body of higher-level organisms, and research is still inconclusive about the abortive nature of these nanoplastics.
Figure 5.7. Microplastic density averaged across individual bottles and lots by brand. Blue bars are densities for “NR + FTIR confirmed particles” (>100 um); orange bars are for “NR tagged particles” (6.5–100 um). Error bars are one standard deviation. Percentages are for the contribution to the total for “NR tagged particles” (6.5–100 um); the contribution of larger particles can be inferred. (This graph is adopted from Mason et al. 2018; permitted to use by Creative Commons.)
Heavy Metal Generation
Heavy metals are added to the environment due to the management of waste where municipality waste is not adequately separated and managed. So directly, it is deposited or disposed of as solid and non-biodegradable waste. Heavy metals in compost products from municipal waste are the crude source (Kupper et al., 2014). The amount of heavy metal in compost is determined by the collection method of the solid waste and the separation of the mix from other metals. Treatment criteria are followed through the legal criteria of the country. A study conducted in Spain showed that 30 samples from different mechanical and biological treatment plants could maintain the legal first level of heavy (quality A) metal concentration such as nickel, chromium, copper, cadmium, and mercury in solid waste; the concentration of lead and zinc was below the legal standard in only four and two plants respectively (Montejo et al., 2015). Another study concluded that mechanically separated waste exceeded the standard level of tolerance compared to the standard maintained in the UK (Farrel & Jones, 2015). Table 1 was adapted and recreated from Wei et al. (2027).
Table 1: Maximum acceptable concentration of metal in different countries (mg/kg dry matter)
Country |
Cadmium |
Copper |
Chromium |
Mercury |
Nickel |
Lead |
Zinc |
Reference |
UK |
1.5 |
200 |
100 |
1 |
50 |
200 |
400 |
BSI-PAS100:2011 |
Italy |
1.5 |
150 |
|
1.5 |
50 |
140 |
500 |
Wei et al. 2009 |
Spain |
0.7 |
70 |
70 |
0.4 |
25 |
45 |
200 |
Spain RD/506 2003 |
Germany |
0.7 |
70 |
70 |
0.5 |
35 |
100 |
300 |
Biowaste Ordinance |
Netherlands |
0.7 |
25 |
50 |
0.2 |
10 |
65 |
5 |
SDU 1991 |
Switzerland |
1 |
100 |
100 |
|
30 |
120 |
400 |
Swiss Federal Council |
California, USA |
39 |
1500 |
1200 |
17 |
420 |
300 |
2800 |
CalRecycle |
USA |
39 |
1500 |
1200 |
17 |
420 |
300 |
2800 |
EPA |
Canada |
3 |
400 |
210 |
0.8 |
62 |
150 |
700 |
CCME |
Heavy Metal Control Strategies
Heavy metal can cause potential risks to human and environmental health. Process control is the primary step to mitigate the disposal of these metals. A downstream control of mitigating steps can reduce the amount of heavy metal in municipal solid waste compost, including separation, advanced mechanical separation, and final product screening to remove heavy metal particles (Shariff & Renella, 2015).
Source separation strategies: Separation is considered one of the most efficient methods of improving the disposed compost in municipal solid waste management. Metals such as Lead, Zinc, Copper, and Cadmium, Chromium, and Nickel can be easily separated by the source separating process. Source-separated waste contains fewer heavy metals than mechanically separated waste, which would be indicative of clean recycling of solid waste. However, this is difficult to conclude based no studies, as these solids tend to contain a heterogeneous mixture of metals and non-metal solid waste.
End-of-the-product separation strategies: To reduce the harmful consequences of heavy metals in soil, countries follow their governmental legal guideline over solid waste management regulations. Table 1 lists the optimum maximum acceptable concentrations of heavy metals in selected countries based on their assessment of the risk of heavy metals from municipality solid waste compost disposed for landfills. These composts are collected from source separation, green waste, and the organic fraction of municipality biowaste reactors with heavy metals compared to natural soil found in backyard soil. The long-term bioavailability of these metals can increase the levels of heavy metals in soil, thus increasing the risk of increased uptake of metals in crops (Wei et al., 2009).
Problems Caused by Biodegradable and Non-biodegradable Waste
Biodegradable and non-biodegradable materials can cause different negative impacts on human health and the environment. Biodegradable waste can cause environmental pollution if the management is not followed, whereas non-biodegradable waste leaves long-term effects on the environment and ecosystem. The effects of this waste are discussed under the following heads:
Air Emission
Air emissions are generated by the waste management system’s incineration plant and air from the waste landfill gases. The fumes generated from open waste burning add hazardous compounds into the air. Waste incineration or control burning practices using advanced and modern incinerators reduce the production of less or no toxic components into the air. But the old incinerators still produce many hazardous emissions like heavy metals and dioxins. An enormous amount of odor-causing gas is emitted from the landfill area of the waste management system, which is poorly managed during the degeneration of organic matter in landfills. The primary gas produced by landfill compost from the degeneration of organic matter is methane (55%), and the carbon dioxide emission amount is 35%. Several other gases also constitute smaller amounts from these landfills and composting plants of the municipality’s solid waste management facility.
Health Impact
The toxic components are generated from biodegradable (short-term and long-term biodegradable components) and non-biodegradable (generated by long-term corrosion and molecule degeneration) and may cause health effects. These health effects may be from direct contact or contaminants flowing through our natural ecosystem.
Persistent organic pollutants from biodegradable waste pose a high risk to human health and the contaminating environment, as these pollutants accumulate through our food web chain. The small animals eating contaminated/bioaccumulated toxins have higher doses of hazardous components than direct contact. For humans, the pollutants they come in contact with either through exposure or the food chain or air-born aerosols may have effects on their nervous system, deteriorate healthy kidneys, and cause cancers. Mismanaged biowaste collected from households and communities—especially excreta and other body liquids—is a severe biohazard and causes the spread of infectious pathogens. For example, acute dermatitis and blood infection can result from direct contact, and eye and respiratory infections might be caused by infected dust particles. Many diseases result from bites of animals eating waste.
Soil and Groundwater
Hazardous substances from biodegradable and non-biodegradable waste may enter the soil. Water passes from contaminated sites, leaching hazardous compounds, fertilizers in agricultural fields, pesticides, etc. These contaminants also seep and carry them into the surface and groundwater. Both soil and groundwater are seriously being polluted due to mismanaged solid biowaste generated by human activities.
Marine Waste
Marine pollution due to plastic waste constitutes a significant danger to marine ecosystems such as fisheries, mangroves, coral reefs, and coastal zones. A major waste in the marine ecosystem is deposited from land-based sources such as persistent organic pollutants, heavy metals from mining, radioactive elements in e-waste, industrial discharge, etc. Plastic waste is a growing concerning material, as it can flow across the world’s oceans. The slow degradation of plastic waste has been drifting on the ocean floor for years. The slow degeneration produces nanoplastic formation, as discussed in the nanoplastic section.
A Louisiana Perspective—Mardi Gras Biodegradable Beads
Mardi Gras, also known as Fat Tuesday, is a festive holiday that is observed annually in New Orleans and cities in southern Louisiana. Due to its size, New Orleans attracts tourists from around the world to participate in all of the historic and decorative parades. Throughout the month of February, parades play a significant role in the celebration where a variety of ornamental beads are thrown into the crowd. Although Mardi Gras was started 300 years ago, it was not until the 1840s that people would throw party favors to the crowd. The traditional colors for Mardi Gras beads, or “throws,” as they are sometimes called, were determined in 1872 to be purple, which stands for power; green, which represents justice; and gold, which represents faith. Traditionally, the beads were glass and then were made out of plastic.
A study from the Ecology Center (Prindle Institute, 2013) reported that each year, Mardi Gras parades used 900,000 pounds of hazardous flame retardants and 10,000 pounds of lead. After the parades, beads readily seep into the surrounding environment when left in gutters, streams, and landfills, having a detrimental impact on human health as well as plant and animal life (The Environmental Impact of Mardi Gras Beads—Prindle Institute). Louisiana State University researchers are creating a biodegradable bead for Mardi Gras festivities.
Learn More: Beyond Biodegradable Mardi Gras Beads (lsu.edu)
Conclusion
Management of biodegradable and non-biodegradable strategies varies from country to country. Every country has a legislative body for controlling the waste management system following the policies and for the enactment of relevant laws. Apart from national policies, these agencies also follow the rules and regulations of international agreements. In many countries, only one government is in authority and responsible for all policies at the federal and national levels. In other countries, several authorities are responsible for different parts of waste management. Sustainable development is the ultimate goal of these organizations. The United Nations formed a more robust organization, “World Economic Forum,” that controls all these international agreements. For more information, please see this link: https://sisu.ut.ee/waste/book/32-sustainable-development-goals-and-international-agreements.
Key Terms
- Hazardous – substances that are flammable, explosive, toxic, or otherwise dangerous.
- e-Waste – electronic products that are not working or useful.
- Bioaerosol – particles released from living organisms of different ecosystems into the atmosphere.
- Heavy metals – metals and metalloids that have high density and toxicity properties even at very low levels of dose in ppb (parts per billion).
- Pesticides – substances used to poison pests. See also pest, fungicide, herbicide, and insecticide.
- Fertilizer – natural or synthetic (man-made) chemicals that can enhance plant growth, development, and productivity.
- Nanoplastic – plastics that are smaller than a micrometer (µm).
- Radioactive waste – Elements made up of atoms whose nuclei are unstable and give off atomic radiation as part of a process of attaining stability.
Review Questions
- Explain or discuss the different types of wastes: e-waste, medical waste, and radioactive waste.
- Discuss how nanoplastic in the environment can cause a threat to the health of animals.
- How do waste management practices cause air emissions?
- Discuss how persistent organic pollutants from biodegradable waste get into the environment.
Critical Thinking / Questions for Discussion
- Discuss or explain how biodegradable materials products are recycled and used in the environment.
- Discuss how marine pollution due to plastic waste constitutes a significant danger to the marine ecosystem.
- How does the U.S. follow governmental legal guidelines over solid waste management regulations?
- Discuss or explain why bioaerosol evaluation is important in the workplace.
References
- Basel Convention on the Control of Transboundary Movements of Hazardous Wastes and Their Disposal (187 signatory countries as of February 2019). Available at: http://www.basel.int/TheConvention/Overview/TextoftheConvention/tabid/1275/Default.aspx
- Bejgarn, S., Macleod, M., Bogdal, C., & Breitholtz, M. (2015). Toxicity of leachate from weathering plastics: An exploratory screening study with Nitocra spinipes. Chemosphere 132, 114e119.
- BSI. (2011). Publicly Available Specification (PAS 100): Specification for Composted Materials.
- Cedervall, T., Hansson, L. A., Lard, M., Frohm, B., & Linse, S. (2012). Food chain transport of nanoparticles affects behaviour and fat metabolism in fish. PLoS One 7, e32254.
- Decision of the Council on the Control of Transboundary Movements of Wastes Destined for Recovery Operations, OECD/LEGAL/0266. Available at: https://legalinstruments.oecd.org/public/doc/221/221.en.pdf
- Farrell, M., & Jones, D. L. (2009). Critical evaluation of municipal solid waste composting and potential compost markets. Bioresource technology, 100(19), 4301–4310.
- Food and Agriculture Organization (2019) SAVE FOOD: Global Initiative on Food Loss and Waste Reduction. Available at: http://www.fao.org/save-food/resources/keyfindings/en/ (accessed in 2019, now archived)
- Hu, D., Shen, M., Zhang, Y., & Zeng, G. (2019). Micro (nano) plastics: An un-ignorable carbon source? Science of the Total Environment, 657, 108–110.
- INTOSAI Working Group on Environmental Auditing. (2016). Auditing waste management. Available at: https://www.environmental-auditing.org/media/5375/wgea-waste-managemen_e.pdf
- O’Connor, D. J., Daly, S. M., & Sodeau, J. R. (2015). On-line monitoring of airborne bioaerosols released from a composting/green waste site. Waste Management, 42, 23–30.
- Pearson, C., Littlewood, E., Douglas, P., Robertson, S., Gant, T. W., & Hansell, A. L. (2015). Exposures and health outcomes in relation to bioaerosol emissions from composting facilities: a systematic review of occupational and community studies. Journal of Toxicology and Environmental Health, Part B, 18(1), 43–69.
- Mendoza, L. M. R., Karapanagioti, H., & Álvarez, N. R. (2018). Micro (nanoplastics) in the marine environment: Current knowledge and gaps. Current Opinion in Environmental Science & Health, 1, 47–51.
- Munari, C., Infantini, V., Scoponi, M., Rastelli, E., Corinaldesi, C., & Mistri, M. (2017). Microplastics in the sediments of terra nova bay (ross sea, Antarctica). Marine pollution bulletin, 122(1–2), 161–165.
- Peeken, I., Primpke, S., Beyer, B., Gütermann, J., Katlein, C., Krumpen, T., … & Gerdts, G. (2018). Arctic sea ice is an important temporal sink and means of transport for microplastic. Nature communications, 9(1), 1505.
- Thompson, R. C., Olsen, Y., Mitchell, R. P., Davis, A., Rowland, S. J., John, A. W., … & Russell, A. E. (2004). Lost at sea: Where is all the plastic? Science, 304(5672), 838–838.
- Lei, K., Qiao, F., Liu, Q., Wei, Z., Qi, H., Cui, S., … & An, L. (2017). Microplastics releasing from personal care and cosmetic products in China. Marine pollution bulletin, 123(1–2), 122–126.
- Jambeck, J. R., Geyer, R., Wilcox, C., Siegler, T. R., Perryman, M., Andrady, A., … & Law, K. L. (2015). Plastic waste inputs from land into the ocean. Science, 347(6223), 768–771.
- Mattsson, K., Hansson, L.A., Cedervall, T. (2015). Nano-plastics in the aquatic environment. Environ. Sci. Process. Impacts 17, 1712e1721.
- Mason, S. A., Welch, V. G., & Neratko, J. (2018). Synthetic polymer contamination in bottled water. Frontiers in chemistry, 407.
- Lu, Y., Zhang, Y., Deng, Y., Jiang, W., Zhao, Y., Geng, J., Ding, L., & Ren, H. Q. (2016). Uptake and accumulation of polystyrene microplastics in zebrafish (Danio rerio) and toxic effects in liver. Environ. Sci. Technol. 50, 12523e12524
- Kashiwada, S. (2006). Distribution of nanoparticles in the see-through medaka (Oryzias latipes). Environ. Health Perspect. 114, 1697e1702.
- Koelmans, A. A., Bakir, A., Burton, G. A., & Janssen, C. R. (2016). Microplastic as a vector for chemicals in the aquatic environment. Critical review and model-supported re-interpretation of empirical studies. Environ. Sci. Technol. 50, 3315.
- Kupper, T., Bürge, D., Bachmann, H. J., Güsewell, S., & Mayer, J. (2014). Heavy metals in source-separated compost and digestates. Waste Management, 34(5), 867–874.
- Montejo, C., Costa, C., & Márquez, M. C. (2015). Influence of input material and operational performance on the physical and chemical properties of MSW compost. Journal of Environmental Management, 162, 240–249.
- Study guide | MOOC: Auditing waste management (ut.ee)
- van Kampen, V., Deckert, A., Hoffmeyer, F., Taeger, D., Brinkmann, E., Brüning, T., … & Bünger, J. (2012). Symptoms, spirometry, and serum antibody concentrations among compost workers exposed to organic dust. Journal of Toxicology and Environmental Health, Part A, 75(8–10), 492–500.
- Xiong, X., Zhang, K., Chen, X., Shi, H., Luo, Z., & Wu, C. (2018). Sources and distribution of microplastics in China’s largest inland lake–Qinghai Lake. Environmental pollution, 235, 899–906.
- Wei, Y., Li, J., Shi, D., Liu, G., Zhao, Y., & Shimaoka, T. (2017). Environmental challenges impeding the composting of biodegradable municipal solid waste: A critical review. Resources, Conservation and Recycling, 122, 51–65.
- World Bank. (2018). What a waste 2.0. A Global Snapshot of Solid Waste Management to 2050. Available at: https://openknowledge.worldbank.org/handle/10986/30317
Substances that are flammable, explosive, toxic, or otherwise dangerous.
Electronic products that are not working or useful.
Waste made up of atoms whose nuclei are unstable and give off atomic radiation as part of a process of attaining stability.
Particles released from living organisms of different ecosystems into the atmosphere.
Plastics that are smaller than a micrometer (µm).
Metals and metalloids that are with high density and toxicity properties even at the very low levels in ppb (parts per billion).
Natural or synthetic (man-made) chemicals that can enhance the plant growth, development, and productivity.
Substances used to poison pests. See also pest, fungicide, herbicide, and insecticide.