Chapter 4 ~ Renewable and Non-renewable Energy Sources
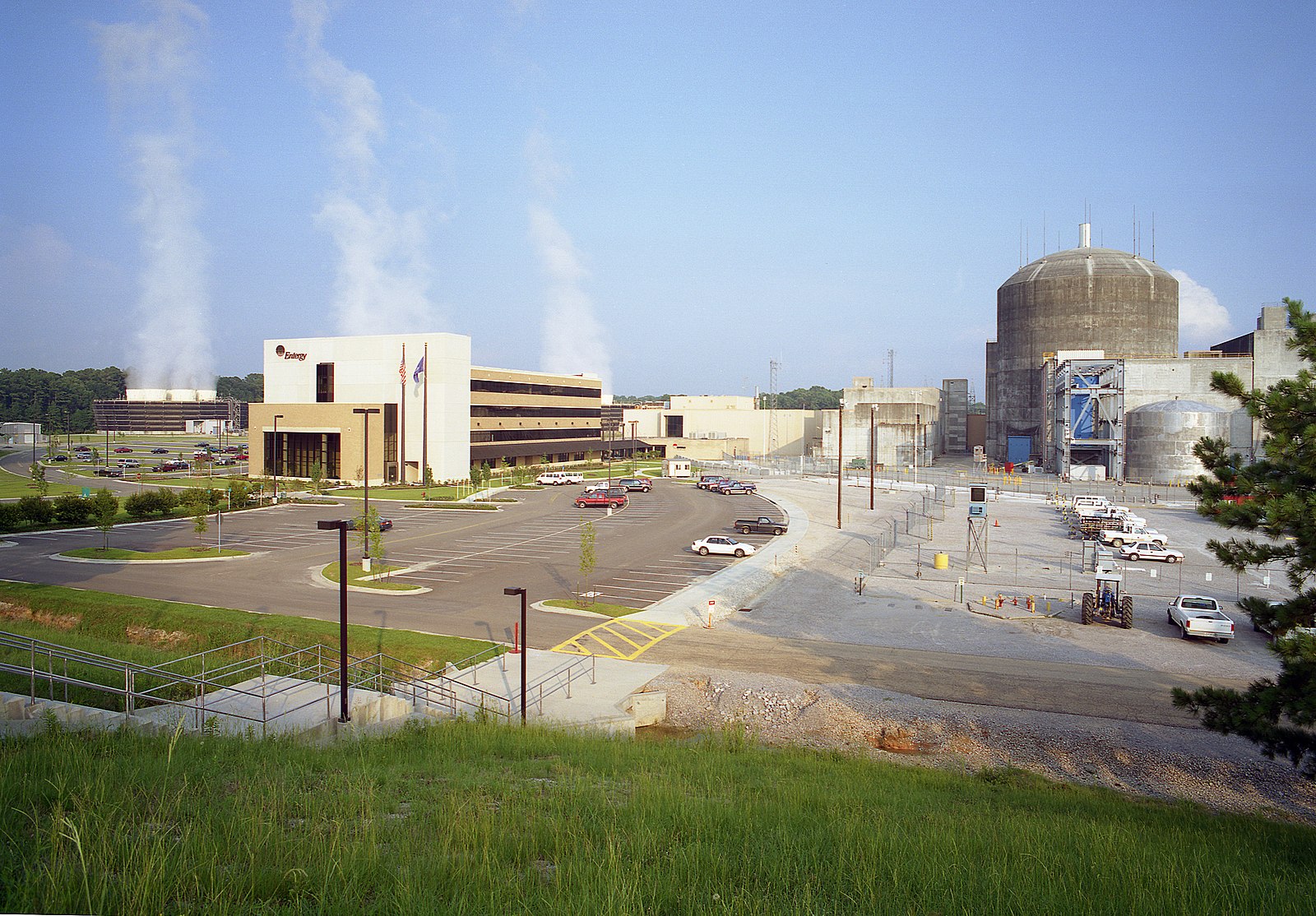
Key Terms
Coal formation, decarbonization, emissions, solar energy, wind energy, oil formation, gas production, energy efficiency, nuclear energy, geothermal energy, natural gas
Learning Objectives
Upon completion of this chapter, students will be able to
- Define energy and explain its properties.
- List specific examples of non-renewable energy sources.
- Describe the types of non-renewable sources utilized for energy extraction and how these sources are formed.
- Describe arguments for non-renewable energy.
- List specific examples of renewable energy sources.
- Describe the advantages and disadvantages of renewable energy sources (i.e., solar, wind, hydroelectric, and geothermal).
- Describe arguments for renewable energy.
- Explain innovative practices of energy implementation in the digital age.
Chapter Overview
- Introduction
- Energy
- Non-renewable Energy Sources
- Renewable Energy Sources
- Energy in the United States
- Energy in Louisiana
- Strategies for Transitioning to Sustainable Energy
- The Fourth Industrial Revolution
Introduction
Living organisms need energy to perform life-sustaining “work” to survive. For nearly all living systems on Earth, the sun is the ultimate source of that energy. Over time, we humans have developed an understanding of energy that has allowed us to harness it for uses well beyond basic survival. The development and evolution of human society are largely attributed to our relationship with energy. The first major advancement in human understanding of energy was the mastery of fire for cooking and heating. Modern civilization is especially dependent on energy, and some of its most distinct characteristics such as population growth, environmental impact, and climate change are all a consequence of energy use. We use energy to heat and light our homes; power our machinery; fuel our vehicles; produce plastics, pharmaceuticals, and synthetic fibers; and provide the comforts and conveniences to which we have grown accustomed in the industrial age. Societal complexity, affluence, and the gap between poor and rich people are all directly related to our level of energy consumption.
Energy sources are central aspects of our modern society, and being able to comprehend and understand them is essential for addressing the global challenges of climate change, energy security, and environmental sustainability and preservation. The world is hungrier than ever for energy as the global human population is growing at a rate of 1.1% per year. Per capita energy consumption today averages just 2.5 kW worldwide. Lifting all of humanity to the current US standard of living by 2100—an average of 9.5 kW per person, probably a conservative projection—thus, means generating more than 51 TW of energy on top of everything we already produce today. To meet such demands, many projects are currently in progress. There are 5,000+ active energy projects in the Middle East and Africa estimated at over USD 2 trillion (ADIPEC 2023 Report).
Energy
Energy is defined as the capacity to do work, and it is fundamental to almost all aspects of life, industry, and technology. It powers our homes, industries, transportation, and more. Energy exists in various forms, including thermal, mechanical, electrical, chemical, hydropower, and nuclear. There are two categories that all energy falls into: kinetic and potential. Kinetic energy refers to types of energy associated with motion. For example, a rock rolling down a hill, the wind blowing through trees, water flowing over a dam, and a cyclist riding a bicycle are just a few examples of kinetic energy. Potential energy is the energy possessed by an object or system due to its position in space relative to another object or system and the forces between the two. Examples include a rock poised at the top of a hill and water stored behind a dam. Some forms of energy are part kinetic and part potential energy. Chemical energy describes the potential of a chemical substance to undergo a chemical reaction and transform other chemical substances; hence it is a form of potential energy. Examples include energy stored in the food you eat and the gasoline that you put in your car.
Fossil fuels (coal, oil, natural gas) have historically been the primary sources of energy. However, they cause significant environmental and climate challenges due to their carbon emissions and other pollutants. In this chapter, energy will be reviewed in two categories: non-renewable energy and renewable energy.
Non-Renewable Energy Sources
Fossil fuel is the term given to an energy source that has a high hydrocarbon content and is found in the Earth’s crust, formed in the geologic past, and can be burned easily to release energy. Fossil fuels were formed from prehistoric plants and animals that lived hundreds of millions of years ago (100–500 million years ago). When these ancient living organisms died, they were quickly buried and subjected to immense pressure from overlying earth materials, including layers of mud, rock, sand, and sometimes surface water bodies such as oceans and lakes.
During the millions of years that passed, the dead plants and animals slowly decomposed in anaerobic (very low to no oxygen) conditions, and their chemical energy became concentrated. The organic compounds that once made up tissues of these organisms were chemically changed under high pressures and temperatures over time. While some fossil fuels are likely in the process of formation today, the amount of time required for usable quantities to form is measured in millions of years, so these fuels will never be available to us. Thus, for all practical purposes, we consider fossil fuels to be finite and a non-renewable resource.
Fossil Fuel Types and Formation
There are three main types of fossil fuels—natural gas, oil, and coal—and the specific type formed depends on the combination of organic matter that was present, how long it was buried, and what temperature and pressure conditions existed when they were decomposing.
Oil and natural gas were created from organisms that lived in water and were buried under ocean or river sediments. Long after the great prehistoric seas and rivers vanished, heat, pressure, and bacteria combined to compress and transform the organic material under layers of silt or shale rock (Figure 4.1). In most areas, a thick liquid called oil formed first, but in deeper, hot regions underground, the transformation process continued until natural gas was formed. Over time, some of this oil and natural gas began working their way upward through the earth’s crust until they ran into rock formations called “caprocks” that are dense enough to prevent them from seeping to the surface. It is from under these caprocks that most oil and natural gas is retrieved today.
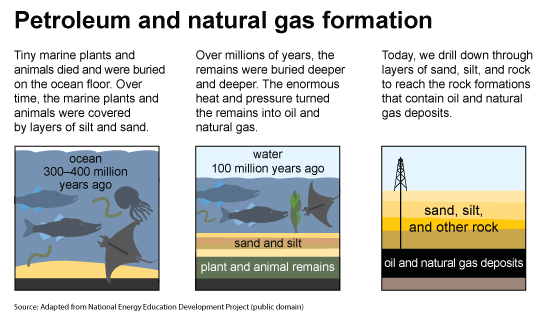
Coal is a fossil fuel that formed from the remains of trees, ferns, and other plants that lived 300 to 400 million years ago (Figure 4.2). In some areas, such as portions of what is now the eastern United States, coal was formed from swamps covered by seawater. The seawater contained a large amount of sulfur, and as the seas dried up, the sulfur was left behind in the coal. Scientists are working on ways to take the sulfur out of coal because when coal burns, the sulfur is released into the atmosphere as an air pollutant (see Chapters 2 and 6). Some coal deposits, however, were formed from freshwater swamps, which had very little sulfur in them. These coal deposits are located largely in the western part of the United States.
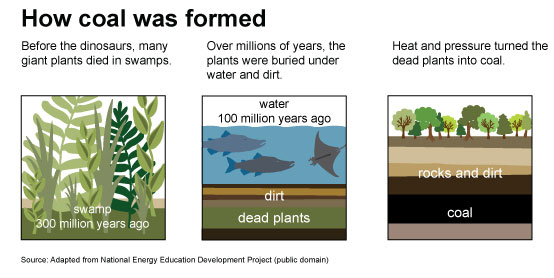
Historically, human prosperity has been directly correlated with energy use. The health and vitality of world societies critically depend on energy, most of which comes from fossil fuels (Figure 4.3). Energy resources, however, are unevenly distributed throughout the world, and so are the consumption rates. Developed regions generally consume far more energy than the developing regions. For example, the United States has only about 5% of the world’s population but constitutes over 20% of the world’s energy consumption. Additionally, developing countries devote a larger proportion of energy consumption to subsistence activities such as growing and preparing food and heating homes. Industrialized nations rely more on mechanized equipment and technology, and therefore, a greater proportion of their energy consumption goes to transportation and industry.
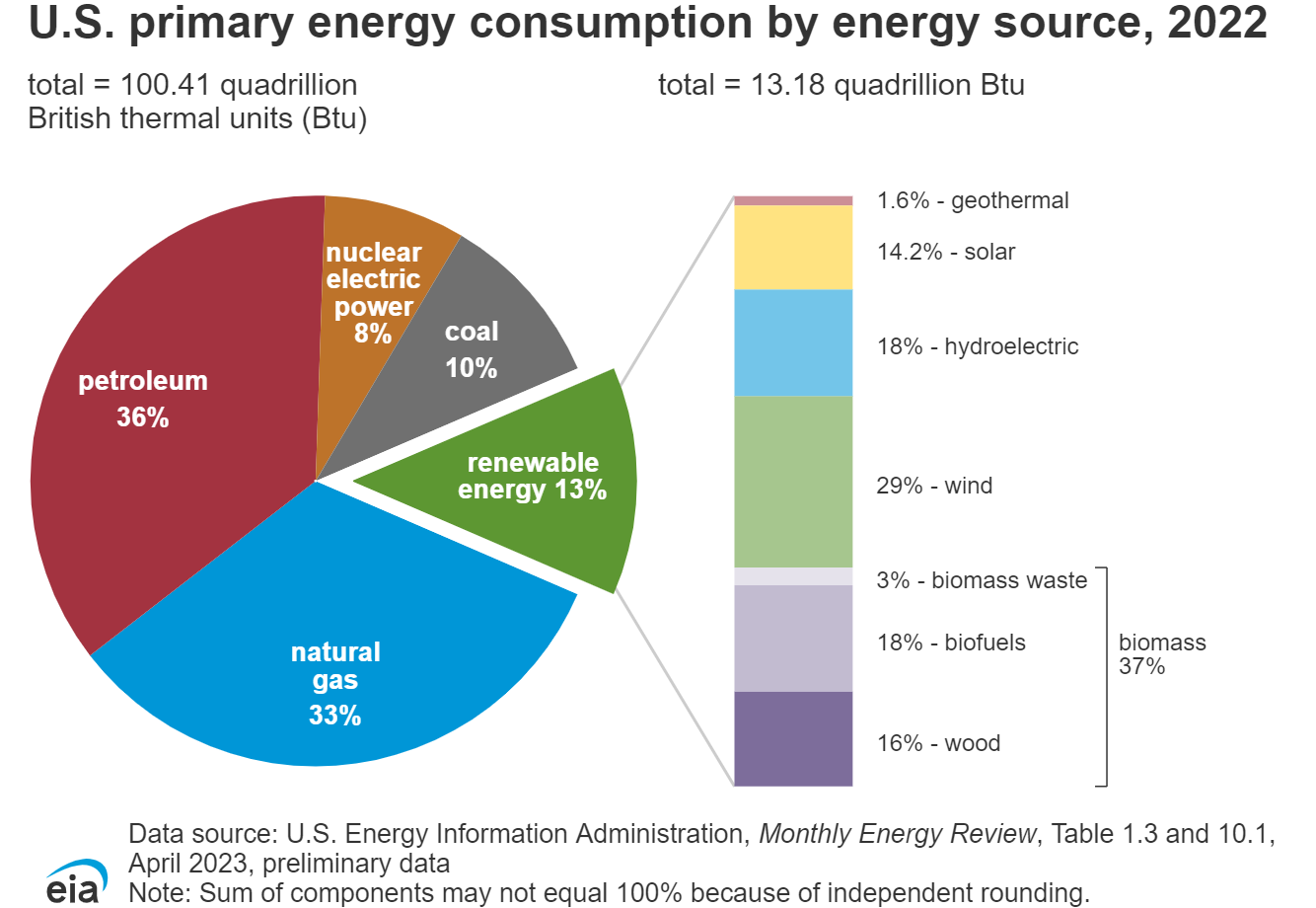
Fossil fuels can be utilized without being converted or transformed into another form of energy; this is referred to as primary energy consumption. In their primary form, fossil fuels can be used for transportation, heating, and cooking or used to generate electricity. The use of electricity is a form of secondary energy consumption. Transforming fossil fuel energy into electricity allows for easier transportation over long distances and application to a variety of uses. Additionally, four major sectors consume energy:
- The industrial sector includes facilities and equipment used for manufacturing, agriculture, mining, and construction.
- The transportation sector includes vehicles that transport people or goods, including cars, trucks, buses, motorcycles, trains, aircraft, boats, barges, and ships.
- The residential sector consists of homes and apartments.
- The commercial sector includes offices, malls, stores, schools, hospitals, hotels, warehouses, restaurants, places of worship, and more.
Each of these sectors also consumes electricity produced by the electric power sector.
Coal
Coal is a combustible black or brownish-black sedimentary rock with a high amount of carbon and hydrocarbons. Coal is classified into four main types, or ranks depending on the types and amounts of carbon present and on the amount of heat energy the coal can produce, including anthracite, bituminous, subbituminous, and lignite. For us to use the potential energy stored in coal, it first must be mined from the ground. This process in itself uses a great deal of resources and has its own environmental impact. Coal then typically undergoes processing to make it suitable for use in coal-fired power plants. Finally, the processed coal is burned in these power plants, and the kinetic energy released from its combustion is harnessed for electricity generation or other purposes.
There are two primary methods of coal mining: strip mining and underground mining. Strip-, or surface-, mining uses large machines to remove the soil and layers of rock known as overburden to expose coal seams. It is typically used when the coal is less than 200 feet underground. Mountaintop removal is a form of surface mining where the tops of mountains are blasted with dynamite and removed to access coal seams. After the mining is finished, the disturbed area can be recovered with topsoil, and the area is replanted. However, the topography of the mountain is permanently altered.
Underground mining, sometimes called deep mining, is used when the coal is several hundred feet below the surface. Some underground mines are thousands of feet deep and extend for miles. Miners ride elevators down deep mine shafts and travel on small trains in long tunnels to get to the coal. The miners use large machines that dig out the coal.
Impacts of Coal Mining and Burning
A majority of the coal mined in the United States (about 66%) is from surface, or strip mines, which leave highly visible impacts at the surface. Strip mining operations generally involve removing soils, rock, and other materials to access shallow deposits of coal and therefore leave permanent scars on the landscape. It also involves the destruction of substantial amounts of forests and other ecosystems, destroying natural habitats and threatening biodiversity (Figure 4.4).
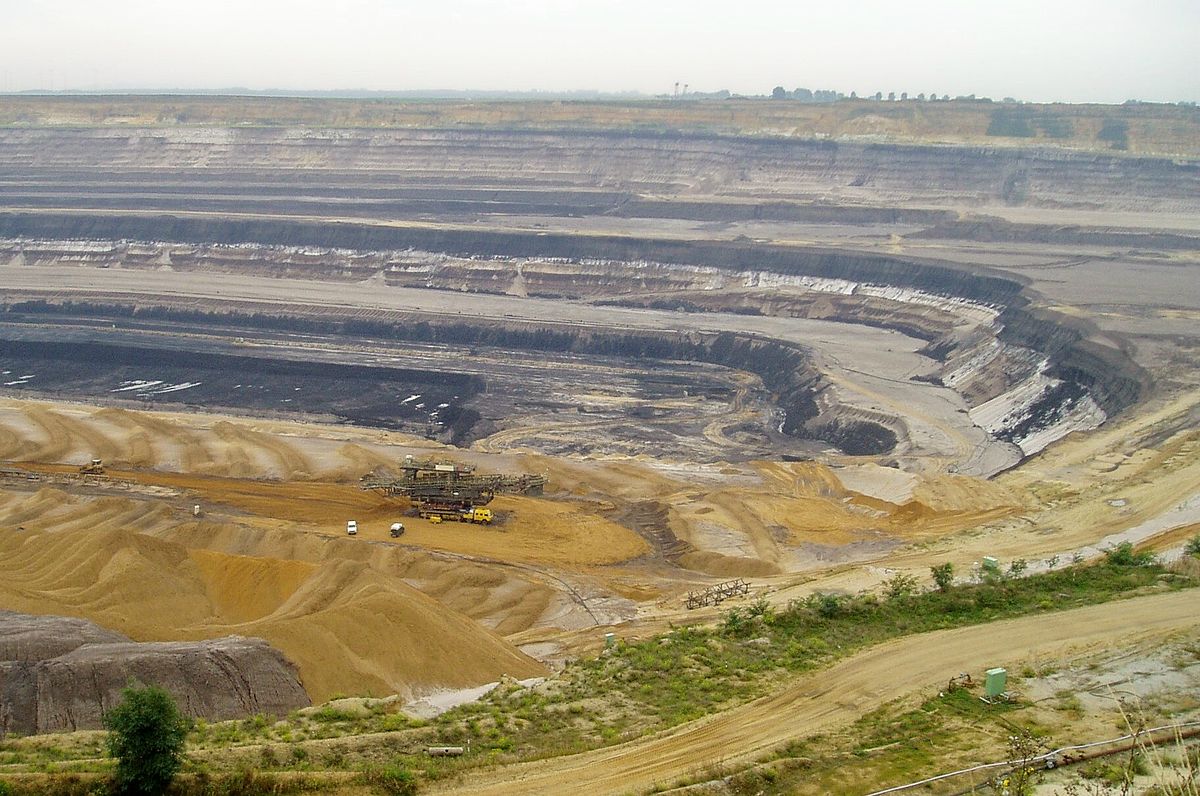
Mountaintop removal, the extreme form of strip mining, has affected large areas of the Appalachian Mountains in West Virginia and Kentucky. The tops of mountains are removed using a combination of explosives and mining equipment, and the material is deposited into nearby valleys. This technique not only alters the landscape (Figure 4.5) but also affects the health and quality of nearby streams by depositing rocks, dirt, and pollutants that can harm aquatic wildlife. While mountaintop removal mining has existed since the 1970s, its use became more widespread and controversial beginning in the 1990s. U.S. laws require that dust and water runoff from areas affected by coal mining operations be controlled and that the area be reclaimed and returned to close to its original condition.
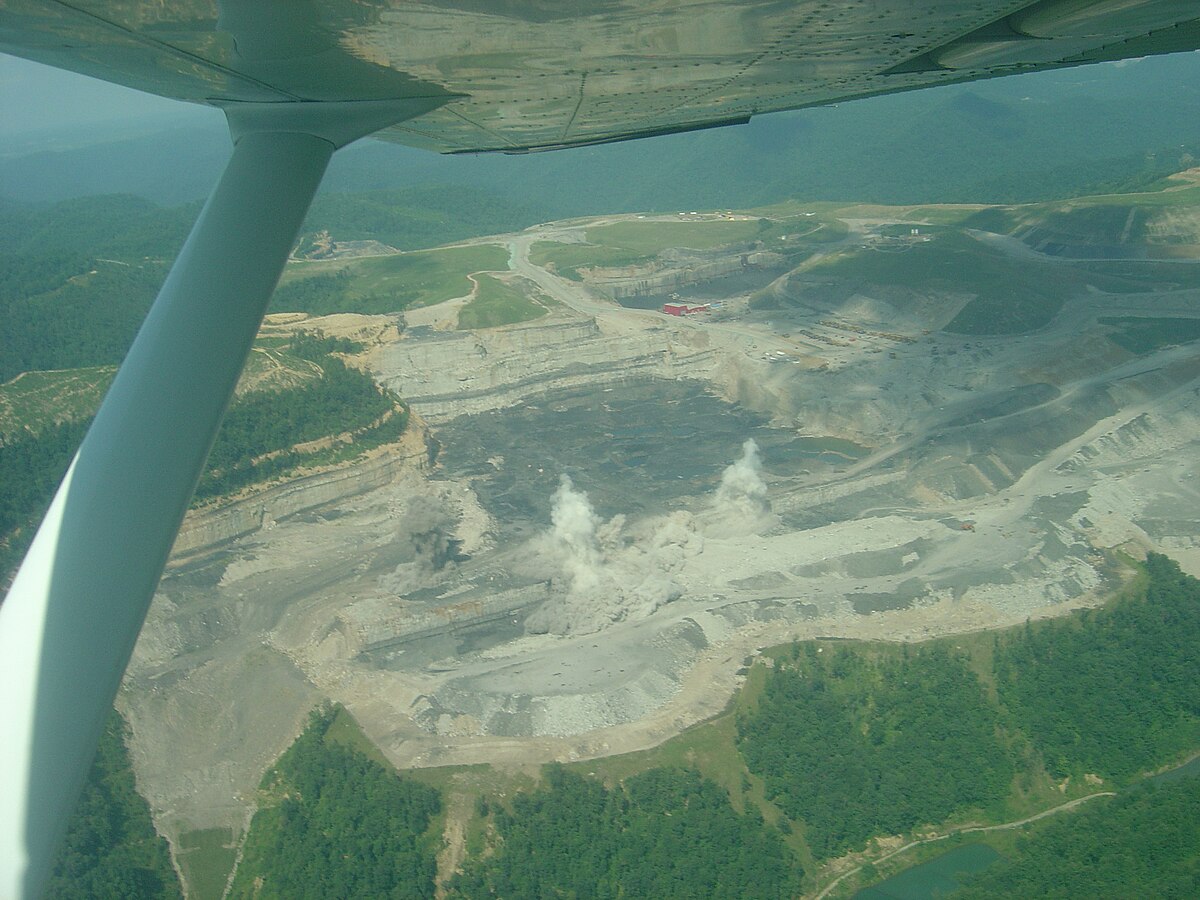
One of the largest environmental impacts of underground mining may be the methane (CH4) gas that must be vented out of mines to make the mines a safe place to work. Methane is a greenhouse gas, meaning that it enhances the greenhouse effect naturally occurring in our atmosphere, and contributes to global warming and global climate change. Its global warming potential, or relative capacity to produce the greenhouse effect, is higher than that of carbon dioxide (see Chapter 8). Other impacts of underground mining include ground collapse above mine tunnels and the draining of acidic water from abandoned mines into nearby streams. Acidic water lowers the pH (resulting in increased acidity), which is detrimental to aquatic organisms. This acid mine drainage has an environmental impact associated with both underground mining and strip mining.
Impacts of Coal Burning on the Environment and Human Health
In the United States and most of the world, most of the coal consumed is used as a fuel to generate electricity. Burning coal produces emissions such as sulfur dioxide (SO2) and nitrogen oxides (NOx) that are associated with acid rain (already discussed in Chapter 2). Carbon dioxide (CO2), another emission resulting from burning coal, is a major greenhouse gas that is associated with global warming (see Chapter 8).
Ash (including fly ash and bottom ash) is a residue created when coal is burned at power plants. In the past, fly ash was released into the air through the smokestack, where it would contribute to particulate matter air pollution. Laws now require that much of the fly ash must be captured by pollution control devices, like scrubbers. In the United States, fly ash is generally stored at coal power plants or placed in landfills. Pollution leaching from ash storage and landfills into groundwater and the rupture of several large impoundments of ash are environmental concerns.
Burning coal produces emissions that also impact human health. Emissions such as sulfur dioxide, nitrogen oxides, and particulates contribute to respiratory illnesses. Particulates also contribute to a condition among coal miners and other coal workers known as coal workers’ pneumoconiosis (CWP) or black lung disease, which results from long exposure to coal dust. Inhaled coal dust progressively builds up in the lungs and is unable to be removed by the body; this leads to inflammation, fibrosis, and in worse cases, tissue death (necrosis).
Coal is the largest source of mercury and also a source of other heavy metals, many of which have been linked to both neurological and developmental problems in humans and other animals. Mercury concentrations in the air usually are low and of little direct concern. However, when mercury enters the water, either directly or through deposition from the air, biological processes transform it into methylmercury, a highly toxic chemical that bioaccumulates in fish and the animals (including humans) that eat fish, as animal bodies lack sufficient excretion pathways for this chemical. This means that the concentration of methylmercury will typically increase throughout an individual’s lifetime if it is present in their food. Methylmercury also biomagnifies in aquatic food chains, meaning that animals at higher trophic levels typically contain more methylmercury than animals at lower trophic levels. Biomagnification is further explained in Figure 4.6.
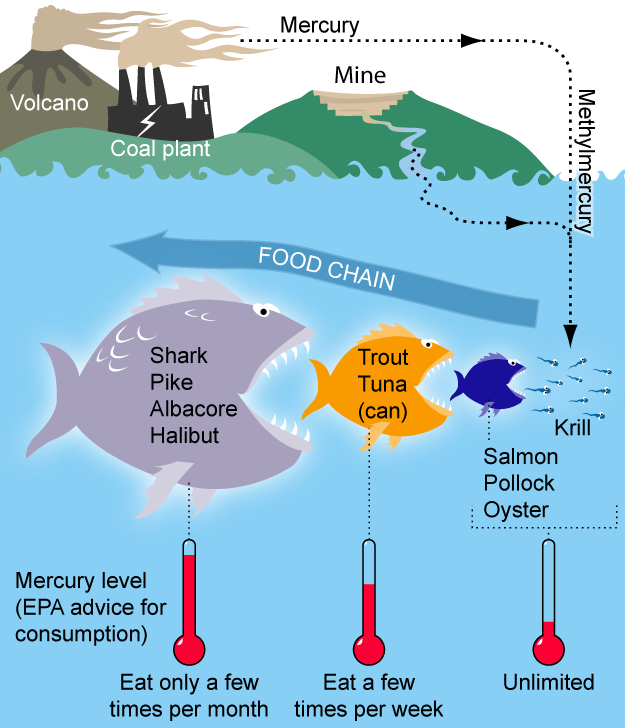
Reducing the Environmental Impacts of Coal Use
Regulations such as the Clean Air Act and the Clean Water Act require industries to reduce pollutants released into the air and water. Below are some actions that have been taken to reduce the negative impacts of coal on human and environmental health:
- Clean coal technology: The industry has found several ways to reduce sulfur, NOx, and other impurities from coal before burning.
- Coal consumers have shifted toward greater use of low-sulfur coal.
- Power plants use scrubbers to clean SO2, NOx, particulate matter, and mercury from the smoke before it leaves their smokestacks. In addition, industry and the U.S. government have cooperated to develop technologies that make coal more energy-efficient so less needs to be burned.
- Research is underway to address emissions of carbon dioxide from coal combustion. Carbon capture & sequestration (CCS) separates CO2 from emissions sources and recovers it in a concentrated stream. The CO2 can then be sequestered, which puts CO2 into storage, possibly underground, where it will remain permanently.
- Reuse and recycling can also reduce coal’s environmental impact. Land that was previously used for coal mining can be reclaimed and used for airports, landfills, and golf courses. Waste products captured by scrubbers can be used to produce products like cement and synthetic gypsum for wallboard.
Oil
Petroleum oil is currently the most widely used fossil fuel and accounts for about one-third of global energy consumption. Unlike coal, which is primarily used as a fuel for electricity generation, oil is primarily used as a fuel for transportation. Oil is also used to manufacture plastics and other synthetic compounds ubiquitous in our everyday life. Crude (unprocessed) oil varies greatly in appearance depending on its composition. It is usually black or dark brown (although it may be yellowish, reddish, or even greenish). In the reservoir, it is usually found in association with natural gas, which being lighter, forms a gas cap over the oil.
Oil is made up of hydrocarbons, which are molecules that contain hydrogen and carbon in various lengths and structures, from straight chains to branching chains to rings. Hydrocarbons contain a lot of energy, and many of the things derived from crude oil like gasoline, diesel fuel, paraffin wax, etc., take advantage of this energy.
Extraction
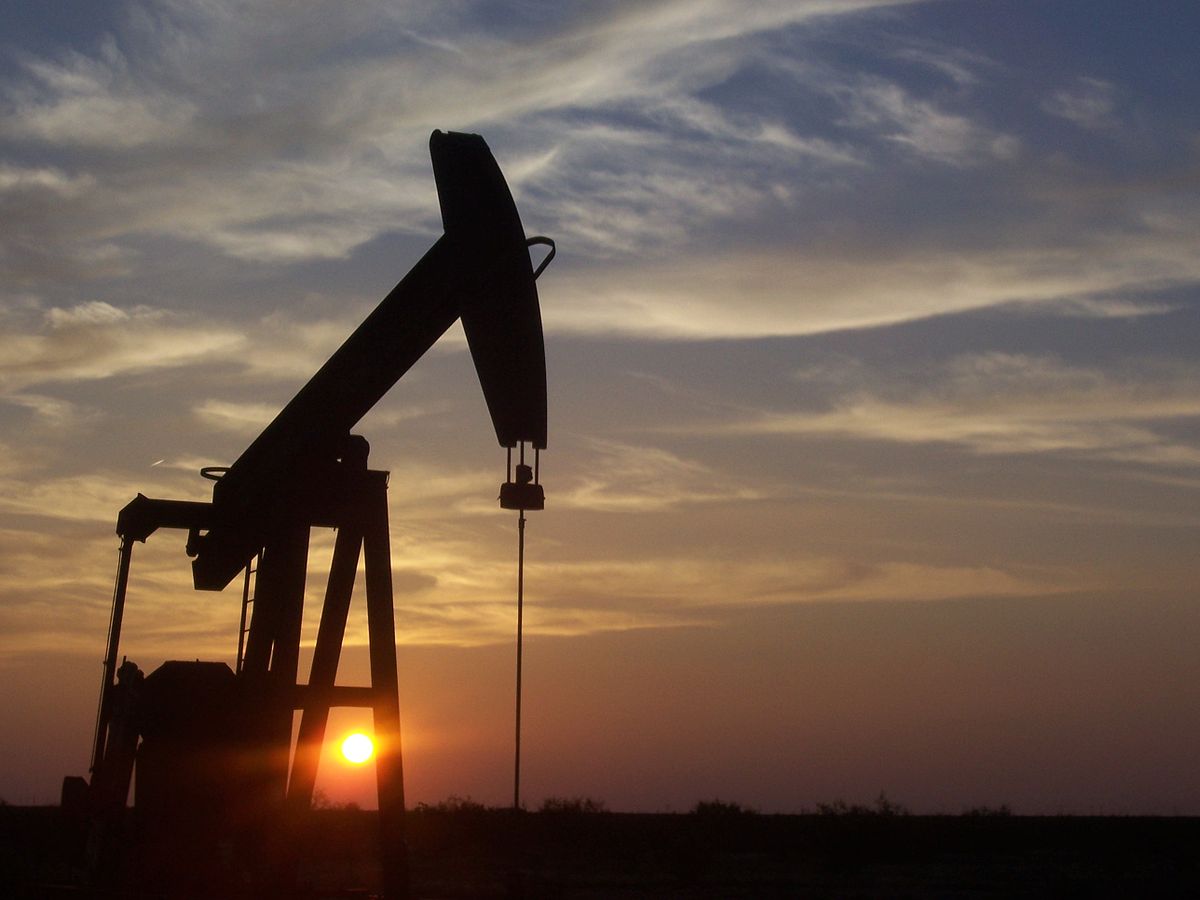
Oil is mainly obtained by drilling either on land (onshore) or in the ocean (offshore) (Figures 4.7 and 4.8). Early offshore drilling was generally limited to areas where the water was less than 300 feet deep. Oil and natural gas drilling rigs now operate in water as deep as two miles. Floating platforms are used for drilling in deeper waters. These self-propelled vessels are attached to the ocean floor using large cables and anchors. Wells are drilled from these platforms, which are also used to lower production equipment to the ocean floor. Some drilling platforms stand on stilt-like legs that are embedded in the ocean floor. These platforms hold all required drilling equipment as well as housing and storage areas for the work crews.
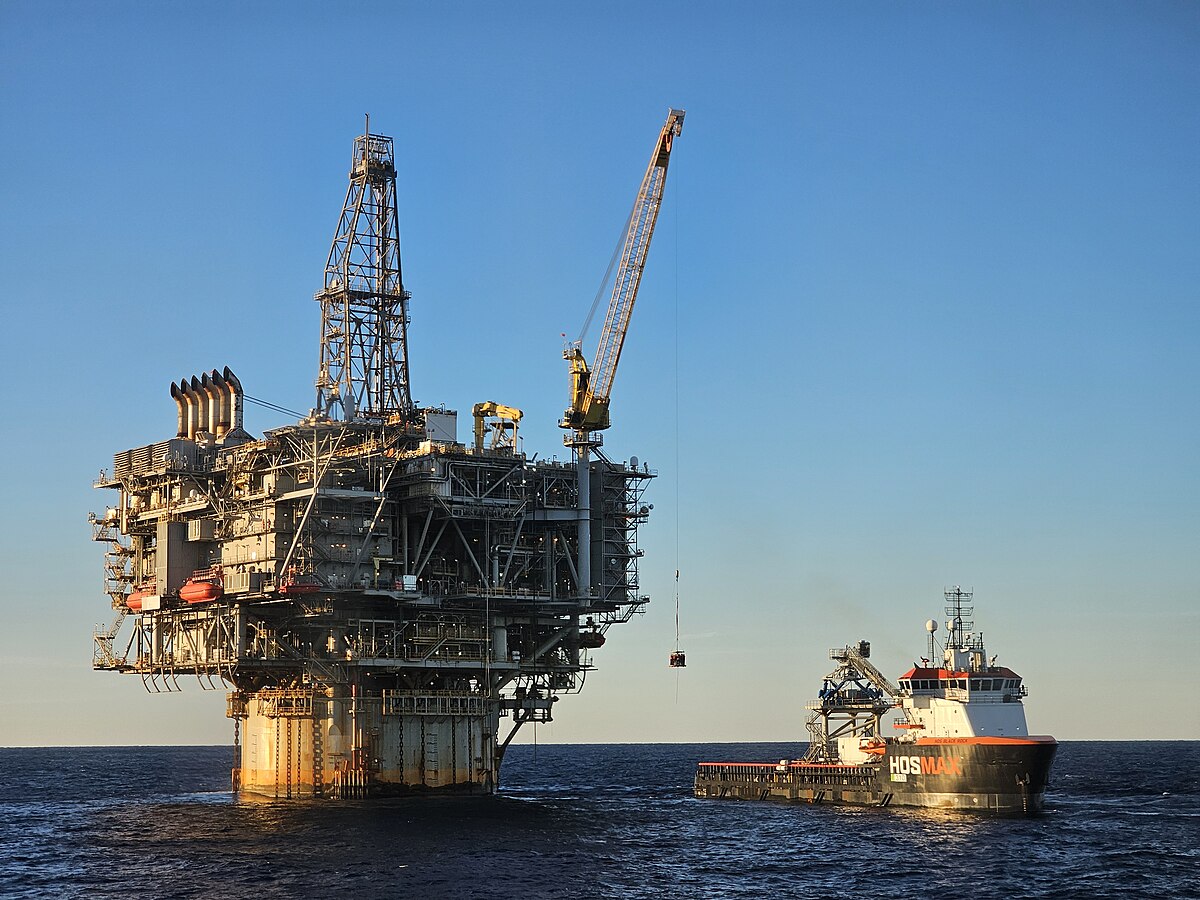
Offshore oil producers are required to take precautions to prevent pollution, spills, and significant changes to the ocean environment. Offshore rigs are designed to withstand hurricanes. Offshore production is much more expensive than land-based production. When offshore oil wells are no longer productive enough to be economical, they are sealed and abandoned according to applicable regulations.
The oil harvested from both offshore and onshore operations must be sent to consumers. Additionally, oil is heavily traded on the international market. Oil can be transported long distances by tanker ship over water or by pipeline over land. Both of these have the potential for leaks and/or spills.
Fracking for Oil
Hydraulic fracturing, informally referred to as “fracking,” is an oil well development process that typically involves injecting water, sand, and chemicals under high pressure into a bedrock formation via the well. This process is intended to create new fractures in the rock as well as increase the size, extent, and connectivity of existing fractures. Hydraulic fracturing is a well-stimulation technique used commonly in low-permeability rocks like tight sandstone, shale, and some coal beds to increase oil flow to a well from petroleum-bearing rock formations (Figure 4.9).
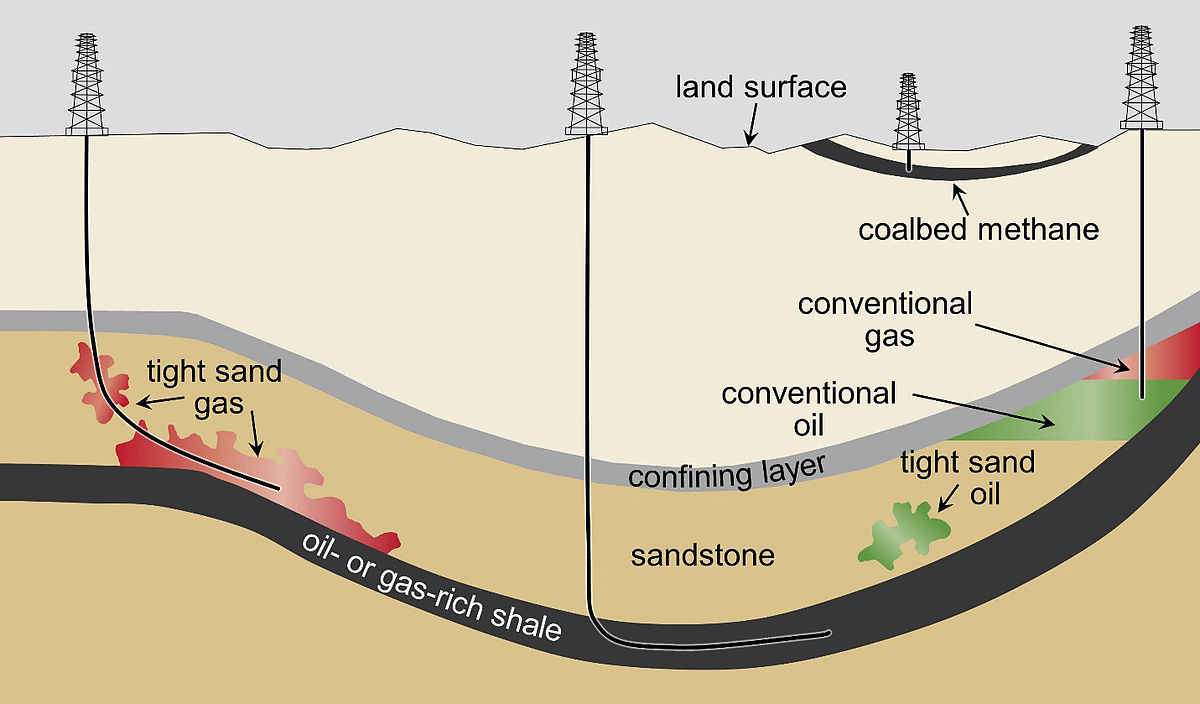
Energy development often requires substantial amounts of water, and hydraulic fracturing is no exception. Water is needed not only for the traditional drilling process but also for the actual fracturing as well. Water is first mixed with chemicals and fine sands, then pumped at extremely high pressure into the shale rock to fracture it, forming pathways for the oil and gas to reach the well. The water is then recovered, along with the oil and gas.
There are concerns regarding the potential contamination of fresh groundwater resources from oil and gas extraction wells that use hydraulic fracturing; either from the petroleum resource being produced or from the chemicals introduced in the fracturing process. Fracking fluid flow back—the fluid pumped out of the well and separated from oil and gas—not only contains the chemical additives used in the drilling process but also contains heavy metals, radioactive materials, volatile organic compounds (VOCs), and hazardous air pollutants such as benzene, toluene, ethylbenzene, and xylene. In some cases, this contaminated water is sent to water treatment plants that are not equipped to deal with some of these classes of contamination.
Environmental Impacts of Oil
Burning petroleum oil products releases emissions such as carbon monoxide (CO), sulfur dioxide, nitrogen oxides, and particulate material, all of which are air pollutants that impact the environment as well as human health (see Chapter 9). Petroleum also emits carbon dioxide, which is a greenhouse gas.
Exploring and drilling for oil may disturb land and ocean habitats. On land, extensive infrastructure such as road networks, transport pipelines, and housing for workers are needed to support a full-scale drilling operation. These can pollute soil and water, fragment habitats, and disturb wildlife.
Human-caused oil spills in rivers and oceans harm ecosystems. Natural oil seepages do occur and may be a significant source of oil that enters the environment globally, but they are slow, small, and spread out over large areas, and the ecosystem has adapted to them. Spills from tankers or well spills have more catastrophic impacts. The quantity of oil spilled during accidents has ranged from a few hundred tons to several hundred thousand tons, but even small spills have been shown to have a great impact on ecosystems.
Oil spills at sea are generally much more damaging than those on land since they can spread for hundreds of nautical miles in a thin oil slick, which can cover beaches with a thin coating of oil. This can kill sea birds, mammals, shellfish, and other organisms it coats. Oil spills on land are more readily containable if a makeshift earth dam can be rapidly bulldozed around the spill site before most of the oil escapes, and land animals can avoid the oil more easily. The amount of oil spilled from ships dropped significantly during the 1990s partly because new ships were required to have a double-hull lining to protect against spills.
Leaks also happen when we use petroleum products on land. For example, gasoline sometimes drips onto the ground when people are filling their gas tanks, when motor oil gets thrown away after an oil change, or when fuel escapes from a leaky storage tank. When it rains, the spilled products get washed into the gutter and eventually flow to rivers and into the ocean. Another way that oil sometimes gets into water is when fuel is leaked from motorboats and Jet Skis.
When a leak in a storage tank or pipeline occurs, petroleum products can also get into the ground, and the ground must be cleaned up. To prevent leaks from underground storage tanks, all buried tanks are supposed to be replaced by tanks with a double lining.
Natural Gas
Crude oil is frequently found in reservoirs along with natural gas. In the past, natural gas was either burned or allowed to escape into the atmosphere. Now, technology has been developed to capture the natural gas and either reinject it into the well or compress it into liquid natural gas (LNG).
Natural gas is predominantly composed of methane (CH4). Some of the gases that are produced along with methane, such as butane and propane (by-products), are separated and cleaned at a gas processing plant. The by-products, once removed, are used in several ways. For example, propane can be used for cooking on gas grills. Natural gas withdrawn from a well may contain liquid hydrocarbons and nonhydrocarbon gases. This is called wet natural gas. The natural gas is separated from these components near the site of the well or at a processing plant. Once the gas is entirely methane, it is then considered dry natural gas and is sent through pipelines to a local distribution company and, ultimately, to the consumer.
A small amount of natural gas is shipped to the United States as LNG. We can also use machines called digesters that turn today’s organic material (plants, animal wastes, etc.) into natural gas through the process of anaerobic decomposition. This process replaces waiting for millions of years for the gas to form naturally. The natural gas produced by these digesters is not a fossil fuel but rather a renewable source of bioenergy.
Fracking for Natural Gas
Conventional natural gas is found in permeable reservoirs, typically composed of sandstone or limestone, where extraction is relatively straightforward because the gas generally flows freely. Unconventional gas is found in rocks with extremely low permeability, which makes extracting it much more difficult. Such gas is extracted by employing so-called unconventional techniques such as hydraulic fracturing (fracking), which has been in use since the late 1940s. In recent decades, fracking technology has greatly improved, and its use has been expanded. The process of fracking for gas is very similar to that of fracking for oil, and the environmental impacts are also similar (see sections above).
As the use of hydraulic fracturing has increased within recent decades, so has the use of natural gas by the electrical generation and manufacturing industries. Most of the natural gas consumed in the United States is produced in the United States. Some are imported from Canada and shipped to the United States in pipelines. Figure 4.10 shows the increase in dry natural gas production in the United States and the resulting increase in natural gas consumption. Meanwhile, we are importing less natural gas as a country. Much of the increase in consumption is due to the construction of numerous natural gas power plants in recent years.
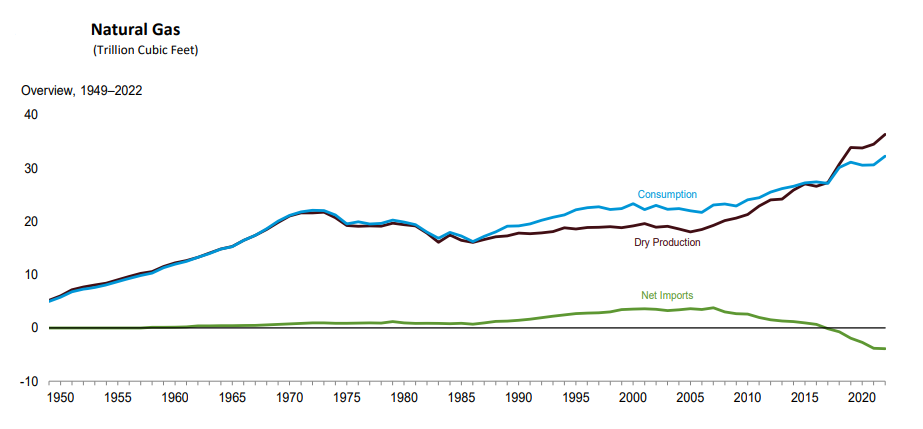
Fossil Fuels and Greenhouse Gases
Fossil fuels are made up mainly of hydrogen and carbon. When burned, the carbon combines with oxygen to create carbon dioxide (CO2). The amount of CO2 produced depends on the carbon content of the fuel. For example, for the same amount of energy produced, natural gas produces about half, and petroleum produces about three-fourths of the amount of CO2 produced by coal. Energy-related CO2 emissions, resulting from the combustion of coal, petroleum, and natural gas, account for about 80% of total U.S. human-caused (anthropogenic) greenhouse gas (GHG) emissions. There are many sources of non-energy CO2 emissions, but those emissions account for a relatively small share of total GHG emissions.
Energy use is largely driven by economic growth and by weather patterns that affect heating and cooling needs. The fuels used in electricity generation also have an impact on the amount of GHG emissions. In the United States, most of the electricity generated comes from coal power plants, and consequently, the majority of the carbon dioxide emission resulting from electricity generation is from coal combustion (Figure 4.11). Although the industrial sector is the largest consumer of energy (including direct fuel use and purchased electricity), the transportation sector emits more carbon dioxide because of its near complete dependence on petroleum fuels. The residential and commercial sectors have lower emission levels (most of which come from fossil energy combustion to produce electricity) than the transportation and industry sectors.
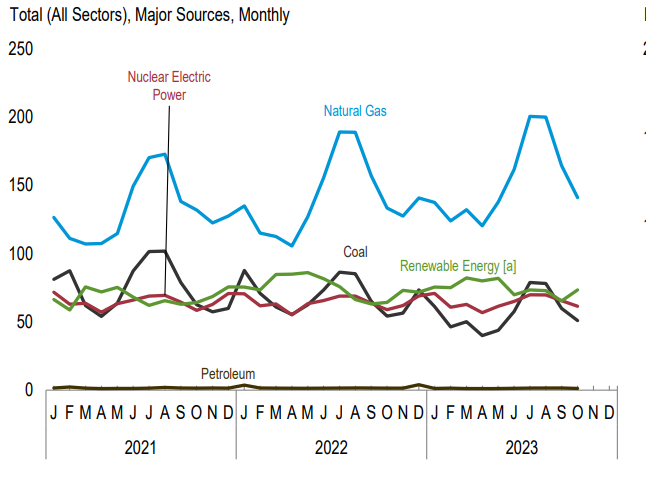
Nuclear Energy
Nuclear energy is energy in the nucleus (core) of an atom. There is enormous energy in the forces that hold protons and neutrons in the nucleus together. Energy is released when those forces are broken. Nuclear energy can be released from atoms by splitting apart the nucleus of an atom to form smaller atoms, a process known as nuclear fission. During nuclear fission, a small atomic particle called a neutron hits the uranium atom and splits it, releasing a great amount of energy in the form of heat and radiation. More neutrons are also released when the uranium atom splits. These neutrons go on to bombard other uranium atoms, and the process repeats itself over and over again. This is called a chain reaction (Figure 4.12). Nuclear power plants use the energy from nuclear fission to produce electricity.
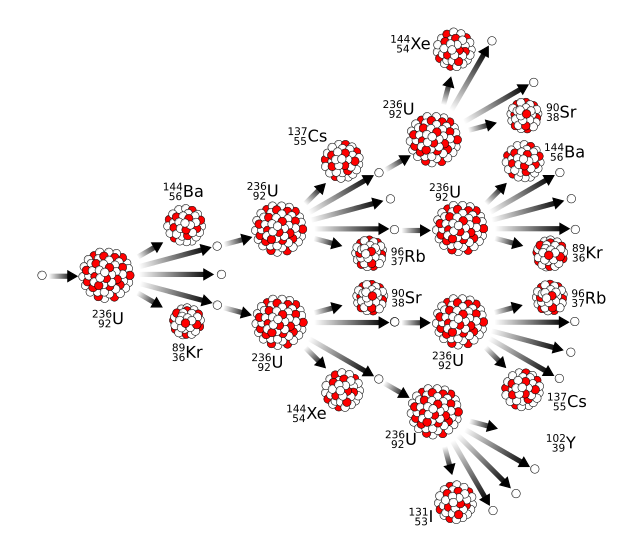
Benefits of Nuclear Energy
By using fission, nuclear power plants generate electricity without emitting air pollutants like those emitted by fossil fuel power plants. This means that financial costs related to chronic health problems caused by air pollutants such as particulate material, carbon monoxide, nitrogen oxides, and ozone, among others, are significantly reduced. In addition, nuclear reactors do not produce carbon dioxide, which means that nuclear energy does not contribute to the global warming problem.
Another benefit of nuclear energy over fossil fuels, especially coal, is that uranium generates far more power per unit weight or volume. This means that less of it needs to be mined, and consequently, the damage to the landscapes is less, especially when compared to the damage that results from coal mining, such as mountaintop removal.
Drawbacks of Nuclear Energy
The main environmental concern related to nuclear power is the creation of radioactive wastes, such as uranium mill tailings, spent (used) reactor fuel, and other radioactive wastes. These materials can remain radioactive and dangerous to human health for thousands of years. Radioactive wastes are classified as low-level and high-level. By volume, most of the waste related to the nuclear power industry has a relatively low level of radioactivity. Uranium mill tailings contain the radioactive element radium, which decays to produce radon, a radioactive gas. Most uranium mill tailings are placed near the processing facility or mill where they come from. Uranium mill tailings are covered with a barrier of material such as clay to prevent radon from escaping into the atmosphere, and they are then covered by a layer of soil, rocks, or other materials to prevent erosion of the sealing barrier.
The other types of low-level radioactive waste are tools, protective clothing, wiping cloths, and other disposable items that get contaminated with small amounts of radioactive dust or particles at nuclear fuel processing facilities and power plants. These materials are subject to special regulations that govern their handling, storage, and disposal so they will not come in contact with the outside environment.
High-level radioactive waste consists of spent nuclear reactor fuel (i.e., fuel that is no longer useful for producing electricity). The spent reactor fuel is in a solid form consisting of small fuel pellets in long metal tubes called rods. Spent reactor fuel assemblies are initially stored in specially designed pools of water, where the water cools the fuel and acts as a radiation shield. Spent reactor fuel assemblies can also be stored in specially designed dry storage containers. An increasing number of reactor operators now store their older spent fuel in dry storage facilities using special outdoor concrete or steel containers with air cooling. There is currently no permanent disposal facility in the United States for high-level nuclear waste.
When a nuclear reactor stops operating, it must be decommissioned. This involves safely removing the reactor and all equipment that has become radioactive from service and reducing radioactivity to a level that permits other uses of the property. The U.S. Nuclear Regulatory Commission has strict rules governing nuclear power plant decommissioning that involve the cleanup of radioactively contaminated plant systems and structures and the removal of the radioactive fuel.
A nuclear meltdown, or uncontrolled nuclear reaction in a nuclear reactor, can potentially result in widespread contamination of air and water. Some serious nuclear and radiation accidents have occurred worldwide. The most severe accident was the Chernobyl accident of 1986 in the then-Soviet Union (now Ukraine), which killed 31 people directly and sickened or caused cancer in thousands more. The Fukushima Daiichi nuclear disaster (2011) in Japan was caused by a 9.0 magnitude earthquake that shut down power supply and a tsunami that flooded the plant’s emergency power supply. This resulted in the release of radioactivity, although it did not directly result in any deaths at the time of the disaster. Another nuclear accident was the Three Mile Island accident (1979) in Pennsylvania, USA. This accident resulted in a near-disastrous core meltdown that was due to a combination of human error and mechanical failure but did not result in any deaths and no cancers or otherwise have been found in follow-up studies of this accident. While there are potentially devastating consequences to a nuclear meltdown, the likelihood of one occurring is extremely small. After every meltdown, including the 2011 Fukushima Daiichi disaster, new international regulations were put in place to prevent such an event from occurring again.
The processes for mining and refining uranium ore and making reactor fuel require large amounts of energy. Nuclear power plants have large amounts of metal and concrete, which also require large amounts of energy to manufacture. If fossil fuels are used for mining and refining uranium ore or in constructing the nuclear plant, then the emissions from burning those fuels could be associated with the electricity that nuclear power plants generate.
Renewable Energy Sources
Energy sources that are more or less continuously made available within a time frame useful to people are called renewable energy. Renewable energy sources are often considered alternative energy sources because, in general, most industrialized countries do not rely on them as their main energy source. Instead, they tend to rely on conventional energy sources such as fossil fuels or nuclear power that are non-renewable. Because of the worldwide energy crisis of the 1970s, dwindling supplies of fossil fuels, and hazards associated with nuclear power, the use of renewable energy sources such as solar energy, hydroelectric, wind, biomass, and geothermal has grown. Renewable energy comes from the sun (considered an “unlimited” or completely renewable supply) or other sources, such as biomass, that can theoretically be renewed at least as quickly as they are consumed (semi-renewable resources).
If used at a sustainable rate, these sources will be available for consumption for thousands of years or longer. Renewable alternatives can be derived from wind, water, solar, or biomass, to name a few. Some renewable energy sources are indirect forms of solar energy because energy from the sun is required to form these sources. Indirect solar energy sources include wind energy, biomass energy, and some forms of water-based energy. The limitations currently associated with most forms of renewable energy include that they are not concentrated, not easily portable, and/or not easy to store.
Energy is an important ingredient in all phases of society. We live in a global society, and access to adequate and reliable energy resources is crucial for economic growth and for maintaining the quality of our lives. However, current levels of energy consumption and production are not sustainable because of the heavy reliance on non-renewable energy sources, which will eventually become depleted. The fuel mix has changed over the years but now is still dominated by fossil fuels. Over 30% of the world’s energy consumption comes from oil, and much of that goes to transportation uses. In 1973, almost 87% of the global energy consumed was from fossil fuels. Today, that value is closer to 80%. Even as the percentage of our energy that comes from fossil fuels is slowly decreasing, it is important to note that our total energy consumption is rapidly increasing.
Why Renewable Energy Sources?
The majority of renewable energy sources, including solar, wind, water, and biomass, can be either directly or indirectly attributed to the sun’s power. The fact that the sun will continue burning for another 4–5 billion years makes it inexhaustible as an energy source for human civilization. With appropriate technology, renewable energy sources can allow for local, decentralized control over their power. Homes, businesses, and isolated communities can use sources such as solar to produce electricity without being near a power plant or being connected to an electrical grid.
In the United States and much of the rest of the world, electricity consumers (homes and businesses) are connected by electrical wires to electricity producers (power plants) through the electrical grid. The grid infrastructure took decades and billions of dollars to establish. Though it would be difficult to generate electricity from coal at home, it is relatively easy to generate electricity from sunlight at a small scale, through the use of photovoltaic cells or wind energy, through the use of wind turbines. This provides important opportunities to deliver renewable energy resources to locations that may lack the financial capital to establish an electrical grid.
Enhanced use of renewable energy sources can also eliminate problems such as oil spills or pipeline leaks. Most renewable energy sources do not pollute the air with greenhouse gas emissions and other air pollutants associated with fossil fuels. This is especially important in combating climate change and improving human health.
Solar Energy
Solar energy is the ultimate energy source driving life on Earth and many human activities. Though only one billionth of the energy that leaves the sun reaches the earth’s surface (Figure 4.13), this is more than enough to meet the world’s energy requirements. Almost all other sources of energy, renewable and non-renewable, are stored forms of solar energy. Solar energy itself is a renewable energy source when energy from the sun is converted to heat or electricity. The difficulties lie in harnessing the energy. Solar energy has been used for centuries to heat homes and water. Modern technology (e.g., photovoltaic cells) has provided a way to produce electricity from sunlight. In 2017, 6% of the renewable energy (<1% of the total energy) consumed in the United States was from solar energy, mostly through the use of photovoltaic cell technology.
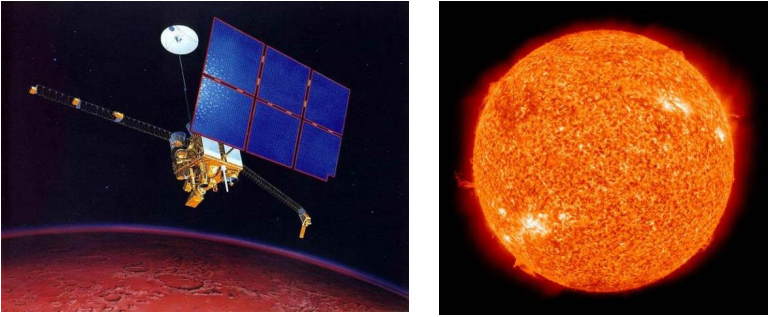
Though solar energy has great potential, there are also some downsides. Solar energy is not evenly distributed across the globe, making some locations better suited to solar energy investment than others. Also, even in locations with great solar potential, solar energy can only be gathered while the sun is shining. This means that little to no energy can be generated at night or on cloudy days. Since sunlight can’t be stored and used on demand (like coal, oil, or even biomass), the challenge of intermittent power can be difficult to overcome. Still, many have found solar energy to be an excellent supplemental source of power, as demonstrated by the increasing popularity of installing solar panels on homes, businesses, and municipal rooftops.
Environmental Impacts of Solar Energy
Solar energy has minimal impact on the environment, depending on where it is placed. The manufacturing of photovoltaic (PV) cells generates some hazardous waste from the chemicals and solvents used in processing, including sodium hydroxide and hydrofluoric acid. Typically, conventional fuel sources, such as fossil fuels, are used to provide energy for PV manufacturing, resulting in the release of greenhouse gases during manufacturing. Ideally, these would be offset by the future use of the solar panel.
Often, solar arrays are placed on roofs of buildings or over parking lots or integrated into construction in other ways. However, large systems and solar farms may be placed on large areas of land. These often occur in deserts, where fragile ecosystems could be damaged by the presence of large solar panels. Some solar thermal systems use potentially hazardous fluids (to transfer heat) that require proper handling and disposal. Concentrated solar systems may need to be cleaned regularly with water, which is also needed for cooling the turbine generator. Using water from underground wells may affect the ecosystem in some arid locations.
Wind Power
Wind power is a renewable energy source that uses the energy of moving air to generate electricity. Winds are caused by differences in atmospheric pressure across the globe. These pressure differentials themselves are largely caused by the temperature differences that result from uneven solar heating across the Earth. In this way, wind power is an indirect form of solar energy. Similar to solar energy, some locations of the Earth’s surface possess greater wind speeds, and therefore a greater capacity for the harvesting of wind energy. Many locations with excellent wind power capacity are found on top of the ocean and are beginning to be utilized through the construction of offshore wind farms.
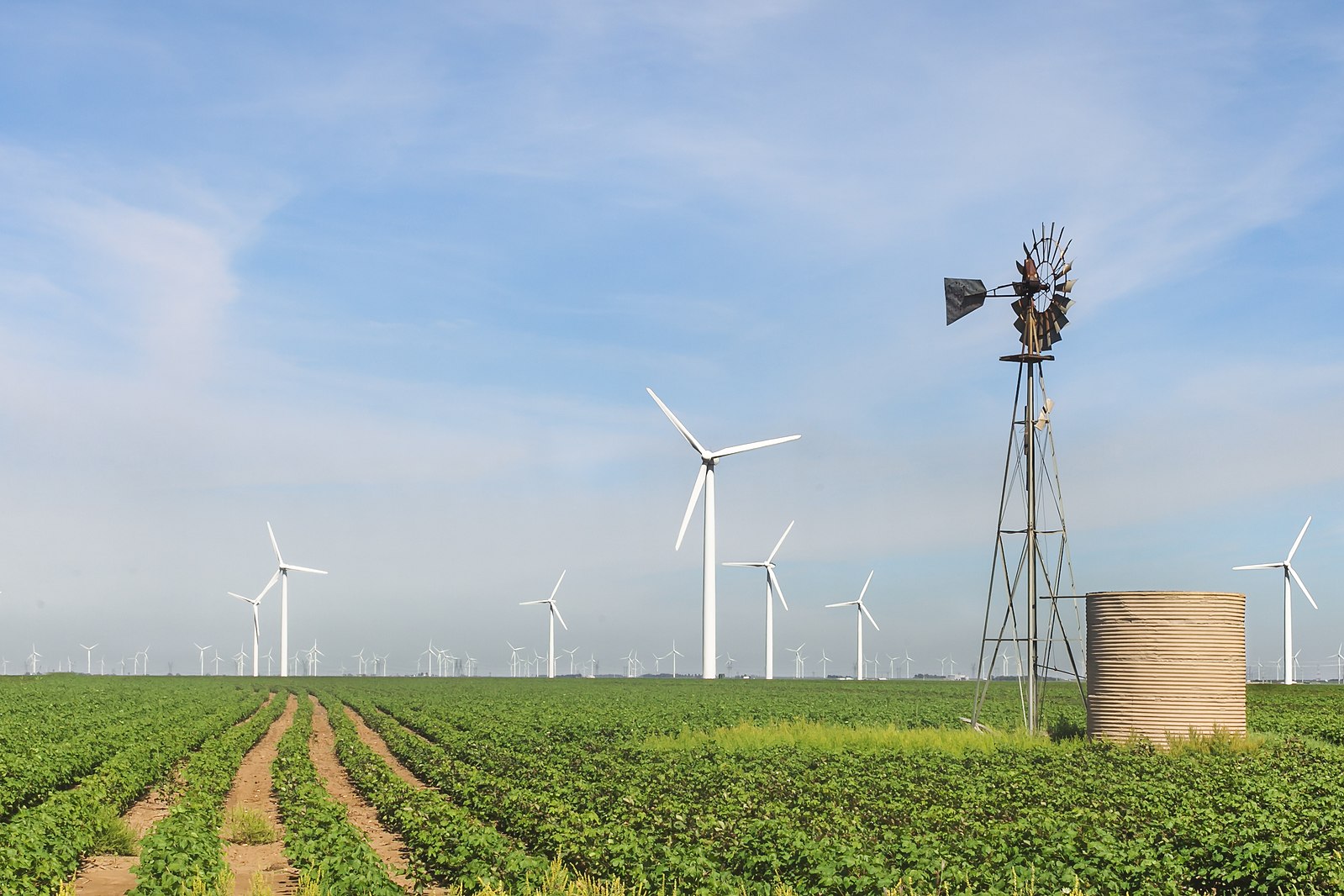
The most common way to collect and transform the wind’s energy into a usable form is through wind turbines. These turbines use blades to collect the wind’s kinetic energy. This technology has been in use for hundreds of years in the form of windmills. While traditional windmills use wind energy to pump water or grind grain, modern wind turbines convert this energy to electricity through the use of a generator. Wind flows over the blades of a turbine, creating lift (similar to the effect on airplane wings), which causes the blades to turn. The blades are connected to a drive shaft that turns an electric generator, which produces electricity.
Wind turbines are becoming a more prominent sight across the United States, even in regions that have less wind potential. Wind turbines do not release emissions that pollute the air or water, and they do not require water for cooling. As of early 2017, the total US wind power installed capacity was 82,183 megawatts (MW). This is up from the 40,181 MW of wind power capacity installed at the end of 2010.
Since a wind turbine has a small physical footprint relative to the amount of electricity it produces, many wind farms are located on crop, pasture, forest land, or coastal areas. They contribute to economic sustainability by providing extra income to farmers and ranchers, allowing them to stay in business and keep their property from being developed for other uses. For example, energy could be produced by installing wind turbines in the Appalachian Mountains of the United States instead of engaging in mountaintop removal for coal mining.
Similar to PV solar systems, wind turbines are practical on a small scale and can be used in remote areas to generate electricity even in the absence of electrical grid infrastructure. Also similar to PV solar systems, it is impossible to store wind and use it on demand. Because of this, wind turbines may be intermittent in their production of power—only producing electricity when the wind is blowing. For this reason, many individuals choose to use them as a supplemental, rather than a primary, electricity source.
Environmental Impacts of Wind Power
Offshore wind turbines on lakes or the ocean may have smaller environmental impacts than turbines on land. Still, wind turbines do have a few environmental challenges. There are aesthetic concerns for some people when they see them on the landscape. A few wind turbines have caught on fire, and some have leaked lubricating fluids, though this is relatively rare.
Wind turbines do produce noise pollution, which can impact both human and animal populations. Locating wind turbines offshore helps to reduce noise pollution in most instances. Additionally, turbines have been found to cause bird and bat deaths particularly if they are located along their migratory path. This is of particular concern if these are threatened or endangered species. There are ways to mitigate that impact, and it is currently being researched.
There are some small impacts from the construction of wind projects or farms, such as the construction of service roads, the production of the turbines themselves, and the concrete for the foundations. However, overall life cycle analysis has found that turbines make much more energy than the amount used to make and install them.
Hydroelectric Power
Hydroelectric power, also known as hydropower, is the second largest source of renewable energy used, next to biomass energy. Similar to wind power, hydropower has been used for hundreds of years as the kinetic energy from moving water was used to turn a mill and grind grain. See an image of a traditional water mill. For most types of hydropower, locations are limited to regions with rivers that are large enough and have a flow strong enough to support a hydropower station. At times when the river is low, there may now be sufficient flow to operate hydropower stations, causing this form of energy to be somewhat limited by both geographical and seasonal factors.
There are three types of hydroelectric power: storage hydropower, pumped-storage hydropower, and run-of-river hydropower. Storage hydropower is one of the major forms of hydropower in the world and consists of dams built across a river to block the flow of river water. The water stored behind the dam contains potential energy, and when released, the potential energy is converted to kinetic energy as the water rushes down. In addition to providing a source of hydroelectric power, the dam also creates a reservoir, or manmade lake, in the area upstream of the dam. Many of the lakes in the Southeastern United States are manmade reservoirs created by hydropower dams, including Lake Lanier, Lake Hartwell, Lake Oconee, and Lake Sinclair.
The pumped-storage hydropower approach involves pumping water from a lower reservoir to a higher reservoir and then allowing it to flow downhill through a turbine, generating electricity. A pumped-storage facility uses energy to pump water from a natural source (ocean, lake, or river) to an upper basin. This process builds a store of potential energy in the water in the upper basin. When energy is needed, water from the upper basin is released through a controlled channel back into the natural source. While this is happening, the water flowing down, out of the upper basin, turns turbines in the channel, which power a generator to produce electricity. Though pumped-storage facilities produce no net energy, they are useful for storing energy to use in times of high demand. The upper basin can be filled during a time when energy is relatively inexpensive and then emptied when energy is costly. Pumped-storage facilities can also be paired with other forms of renewable energy, such as solar or wind, to store energy from these intermittent sources.
Run-of-river hydropower is an alternative approach to hydropower that is considered less disruptive than storage hydropower facilities. It involves diverting a portion of the river’s water through a pipe or channel containing turbines to power a generator and produce electricity. This water is then returned to the river. The largest environmental benefit to run-of-river systems is that they do not create a large reservoir of water above the dam but allow the river to flow at its more natural pace.
The U.S. Department of Energy Office of Energy Efficiency and Renewable Energy
Follow this link to diagrams of a large hydropower system, a run-of-river hydropower facility, and a pumped storage hydropower facility: Types of Hydropower Plants.
Environmental Impacts of Hydropower
Hydropower is a renewable source of energy since it does not directly produce emissions of air pollutants, it consumes no non-renewable fuel sources, and the source of power is constantly regenerated. However, hydropower dams, reservoirs, and the operation of generators can have serious environmental impacts. A dam that is used to create a reservoir or to divert water to a run-of-river hydropower plant can obstruct the migration of fish to their upstream spawning areas in areas where salmon must travel upstream to spawn, such as along the Columbia River in Washington and Oregon. Turbines kill and injure some of the fish that pass through the turbine, though prevention of this is attempted in most facilities. This problem has been partially alleviated in some systems by using fish ladders that help the salmon get up the dams.
Storage hydropower systems are typically the most impactful of all forms of hydropower through their creation of a reservoir. This action destroys the terrestrial ecosystem that previously inhabited the reservoir area and impacts populations of plants and animals on the adjacent land, as food sources and migration paths are disrupted. Construction of reservoirs may cause natural areas, farms, and archeological sites to be covered and force populations to relocate, resulting in the loss of scenic rivers. The construction of the Three Gorges Dam on the Yangtze River in China caused the relocation of over 1 million residents.
Even downstream of the dam, environmental impacts are felt. A reservoir and operation of the dam can affect the natural water habitat due to changes in water temperatures, chemistry, flow characteristics, and silt loads, all of which can lead to significant changes in the ecology and physical characteristics of the river upstream and downstream. Carbon dioxide and methane may also form in reservoirs where water is more stagnant than it would have been in a flowing river and be emitted into the atmosphere. The exact amount of greenhouse gases produced from hydropower reservoirs varies significantly by location and even by season. If the reservoirs are located in tropical and temperate regions, including the United States, those emissions may be equal to or greater than the greenhouse effect of the carbon dioxide emissions from an equivalent amount of electricity generated with fossil fuels (EIA 2011, p. 333).
Geothermal Energy
Geothermal energy uses heat from the Earth’s internal geologic processes to produce electricity or provide heating. The subsurface temperature of the Earth provides an essentially endless energy resource. The energy harvested in a geothermal power plant is the same energy that forms geysers and hot springs. The heat from the Earth’s core continuously flows outward. Sometimes the heat, as magma, reaches the surface as lava, but it usually remains below the Earth’s crust, heating nearby rock and water—sometimes to levels as hot as 370°C (See Chapter 1, Figure 1.3). When water is heated by the earth’s heat, hot water or steam can be trapped in permeable and porous rocks under a layer of impermeable rock, and a geothermal reservoir can form.
A geothermal system requires heat, permeability, and water. To develop electricity from geothermal resources, wells are drilled in a location with high geothermal potential. This is typically a region containing naturally superheated groundwater. Groundwater percolates down through cracks in the subsurface rocks until it reaches rocks heated by underlying magma, and the heat converts the water to steam. Many areas with strong seismic activity, including earthquakes and volcanoes, also possess high geothermal potential. Examples include the country of Iceland, many regions of California, and the North American Pacific Coast. According to the World Energy Council, the total geothermal installed capacity was 83,400 MW by the end of 2015, with 21,000 MW in the US alone.
Geothermal wells bring the superheated water or steam to the surface, where its heat energy is converted into electricity by a generator at a geothermal power plant (Figure 4.15). Wells can also be dug to tap the steam reservoir and bring it to the surface to drive turbines and produce electricity. Geothermal energy can be used for electricity production, commercial, industrial, and residential direct heating purposes, and efficient home heating and cooling through geothermal heat pumps.
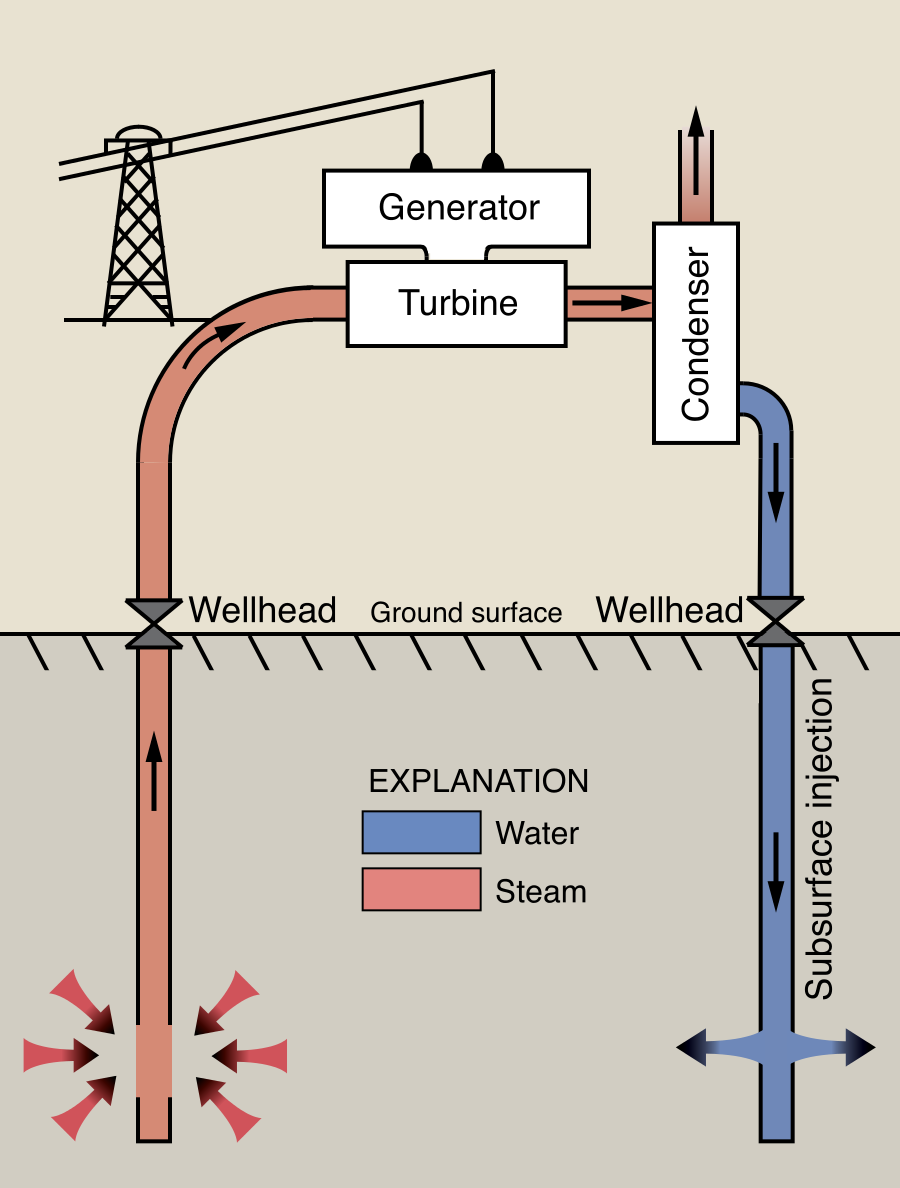
Geothermal Energy at Southeastern Louisiana University
Southeastern Louisiana University was the first higher education institution in Louisiana to develop a hybrid geothermal system to provide electricity to its campus. A pond loop system is used to power two university residence halls, Ascension Hall and Twelve Oaks Hall. The pond is located approximately 1 mile north of the residence halls at the Sustainability Center. To learn more about the system setup and to view a schematic of the hybrid system, visit Geothermal Energy at Southeastern Louisiana University.
Environmental Impacts of Geothermal Energy
The environmental impact of geothermal energy depends on how it is being used. Direct use and heating applications have almost no negative impact on the environment. Geothermal power plants do not burn fuel to generate electricity, so their emission levels are very low. Some carbon dioxide (CO2) and methane (CH4) gas are emitted but to a much smaller degree than the combustion of fossil fuels or biomass. Very small quantities of other gases including ammonia and hydrogen sulfide can also be produced. To help mitigate emissions impacts, geothermal plants use scrubber systems to clean the emissions of the hydrogen sulfide that is naturally found in deep steam and hot water. They emit 97% less acid rain–causing sulfur compounds than are emitted by fossil fuel plants.
Even though geothermal energy is renewable, not every plant built to capture this energy will be able to operate indefinitely because the energy relies on groundwater recharge. If the heated water is used faster than the recharge rate of groundwater, the plant will eventually run out of water. The Geysers, a famous geothermal power plant in California, started experiencing this, and operators responded by injecting treated municipal wastewater into the ground to replenish the supply. Also, patterns of geothermal activity in the Earth’s crust naturally shift over time, and an area that produces hot groundwater now may not always do so. The water of many hot springs is laced with salts and minerals that can corrode equipment, shorten the lifetime of plants, and increase maintenance costs.
Electrical power is restricted to regions where energy can be tapped from naturally heated groundwater, but most areas of the world are not rich in naturally heated groundwater. Engineers are trying to overcome this by drilling deeply into dry rock, fracturing the rock, and pumping in cold water, which becomes heated and drawn up through an outlet well and used to generate power. However, this approach is said to trigger minor earthquakes.
Energy in the United States
Energy in the United States is a crucial aspect of its economy, society, and environment. The U.S. is one of the world’s largest consumers and producers of energy. Historically, it has heavily relied on fossil fuels, but there is an ongoing transition toward a more diverse and sustainable energy portfolio (Figure 4.16). The energy landscape and panorama in the United States is evolving almost exponentially, with a focus on transitioning to a more sustainable, continuous, uninterrupted, diverse, and resilient energy system. This energy scene is currently ongoing, while humanity is addressing climate change and promoting economic growth. The public and private business sectors and community collaborations, robust technological innovations, effective policy support, and accurate public awareness will continue to play significant roles in shaping the future of energy demand and consumption in the United States.
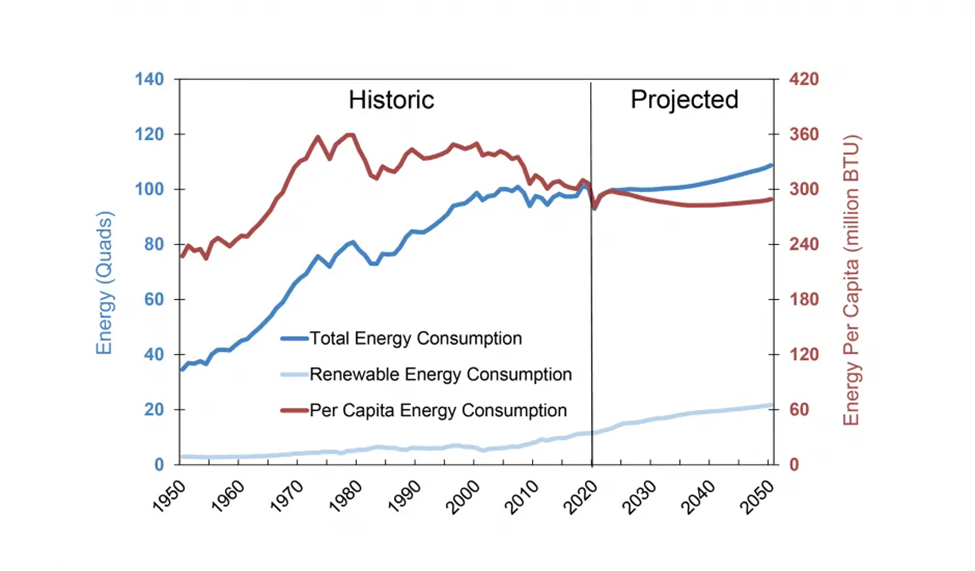
With less than 5% of the world’s population, the U.S. consumes almost 17% of the world’s energy and accounts for 16% of world GDP. In comparison, the European Union has 6% of the world’s population, uses 10.4% of its energy, and accounts for 16% of its GDP, while China has 18% of the world’s population, consumes 25% of its energy, and accounts for 18% of its GDP. Each day, U.S. per capita energy consumption includes 2.5 gallons of oil, 8.86 pounds of coal, and 246 cubic feet of natural gas. Residential daily consumption of electricity is 12 kilowatt-hours (kWh) per person. In 2021, total U.S. energy consumption decreased by 3.1% from 2019 peak levels.
According to current Department of Energy (DOE) estimates, 75% of U.S. energy will come from fossil fuels in 2050, which is widely inconsistent with IPCC carbon reduction goals. Renewable energy consumption is projected to increase annually at an average rate of 2.1% between 2021 and 2050, compared to 0.4% growth in total energy use. Residential photovoltaics are projected to grow annually by 6%. At these rates, renewables would provide 20% of U.S. energy consumption in 2050, compared to 12.5% today. In 2021, for the second time since tracking began, the U.S. exported more oil (8.63 million barrels per day) than was imported (8.46 million barrels per day) and is also expected to be a net exporter in 2050.
Historically, the U.S. has been heavily reliant on fossil fuels, including coal, oil, and natural gas, for its energy needs. In recent years, there has been a significant increase in the use of renewable energy sources, particularly wind and solar, as well as a growth in natural gas usage due to its relatively cleaner nature compared to coal and oil. Renewable energy has been growing rapidly in the U.S., driven by falling costs, policy incentives, and increasing public awareness of climate change. Wind and solar power have seen substantial growth and are now major contributors to the U.S. electricity grid. Hydropower, geothermal, and biomass also contribute to the renewable energy portfolio.
Natural gas has become a dominant source of energy in the U.S., surpassing coal as the leading source of electricity generation. The shale gas boom, driven by advancements in hydraulic fracturing (fracking), has significantly increased domestic natural gas production. Coal consumption has declined due to environmental concerns, increased competition from natural gas and renewables, and a shift toward cleaner energy sources. Many coal-fired power plants have been retired or converted to natural gas to reduce carbon emissions. The U.S. has a significant number of nuclear power plants, providing a substantial portion of the country’s electricity. However, nuclear energy’s growth has been slow due to concerns about safety, nuclear waste management, and high costs.
Improving energy efficiency is a key focus to reduce overall energy consumption and decrease greenhouse gas emissions. This involves adopting energy-efficient technologies, appliances, and industrial processes. The U.S. has made efforts to enhance energy independence by increasing domestic production of oil, natural gas, and renewable energy, thereby reducing reliance on energy imports. Addressing climate change and reducing carbon emissions are major priorities, driving the transition toward cleaner and more sustainable energy sources. Federal, state, and local governments have enacted policies and regulations to encourage renewable energy adoption, energy efficiency, and carbon reduction targets.
Energy in Louisiana
The energy sector in Louisiana continues to evolve, balancing traditional fossil fuel-based industries with a growing emphasis on renewable energy and sustainability. The state’s strategic location, ample energy resources, and ongoing initiatives to diversify its energy mix position Louisiana to play a vital role in the United States’ energy landscape.
Louisiana, which is in the southern region of the United States, has a diverse energy landscape that plays a significant role in both the state and the nation’s energy portfolio. The state is known for its abundant fossil fuel resources, particularly natural gas, and oil, but it has also been making strides in renewable energy development. An overview of the energy map of the state of Louisiana is multistage from Natural Gas and Crude Oil production to Research and Development (including related policy).
Today, Louisiana faces unique challenges when it comes to energy sustainability. The state is a major producer of oil, natural gas, and other fossil fuels, which have historically been a significant part of the state’s economy. However, this dependence on fossil fuels has also made the state vulnerable to fluctuations in global energy prices, as well as environmental risks such as oil spills and other forms of pollution.
In recent years, Louisiana has taken steps to diversify its energy mix and promote sustainability. For example, the state has invested in wind and solar power and is home to several large-scale renewable energy projects. Additionally, the state has implemented energy efficiency programs that aim to reduce energy consumption and save money for businesses and homeowners.
Despite these efforts, there is still much work to be done to ensure energy sustainability in Louisiana. The state continues to face challenges related to environmental degradation, coastal erosion, and other issues that threaten the long-term health of the state’s energy resources. To address these challenges, policymakers and energy industry leaders must work together to promote responsible energy development, invest in renewable energy infrastructure, and support initiatives that promote energy efficiency and conservation. There are several factors impacting energy in the state of Louisiana. A few key factors are briefly described below.
Strategies for Transitioning to Sustainable Energy
Transitioning to sustainable energy is a multidimensional and complex effort that involves technological advancements, policy reforms, behavioral changes, environmental preservation policies and best practices, and global collaboration. It is a key component of moderating climate change and ensuring a sustainable and equitable future for all. Additional key considerations when transitioning to sustainable energy include decarbonization; increased efficiency and conservation efforts; energy access and equity, innovation, and technology; policy regulations; and educating the general public.
Decarbonization refers to shifting from fossil fuels to low or zero-carbon energy sources. This process is essential and in demand to reduce greenhouse gas and other carbon footprint pollutants emissions. The decarbonization process will greatly help humanity to combat climate change. Efficiency and conservation will enhance energy efficiency and promote conservation efforts that are critical aspects of a sustainable energy transition, reducing overall energy demand. Energy access and equity support justifiable access to sustainable energy for all, including supplying power to underserved and remote regions, which is crucial for socioeconomic development and poverty alleviation.
Innovation and technology encourage research, development, and deployment of advanced technologies and are vital to improving the efficiency and cost-effectiveness of sustainable energy solutions. Implementing supportive policies, incentives, and regulations at local, national, and global levels is necessary to drive the adoption of sustainable energy and create a conducive environment for investment and innovation. Raising awareness about the benefits of sustainable energy and educating the public on energy conservation practices are important for fostering a culture of sustainability. Lastly, investments are being made in modernizing the electric grid to accommodate the integration of renewable energy sources and improve grid reliability and resilience.
The Fourth Industrial Revolution (4IR)
The Fourth Industrial Revolution is having a significant impact on energy in Louisiana, as it is changing the way that energy is produced, distributed, and consumed. There are a few reasons that the 4IR is impacting energy in Louisiana.
The 4IR is bringing new digital technologies to the energy sector, including the Internet of Things (IoT), artificial intelligence (AI), and blockchain technology. These technologies are enabling greater automation and optimization of energy systems, leading to improved efficiency and lower costs. The 4IR is driving the adoption of renewable energy sources such as solar and wind power. Louisiana has significant potential for renewable energy, particularly in offshore wind and solar, and the 4IR is helping to make these sources more accessible and cost-effective. The 4IR is also driving innovation in energy storage technologies such as batteries and pumped hydro. These technologies are critical for enabling the integration of renewable energy sources into the grid, as they can help balance supply and demand and provide backup power during times of peak demand. The 4IR is enabling the development of smart grids, which are more efficient and resilient than traditional grids. Smart grids use advanced sensors and analytics to monitor and optimize energy flows, leading to lower costs, reduced emissions, and improved reliability.
The 4IR is also driving the adoption of electric vehicles (EVs), which are becoming more cost-effective and practical as battery technology improves. Louisiana is investing in EV infrastructure to support the adoption of these vehicles, which can help to reduce emissions from transportation and improve air quality. The 4IR is transforming the energy sector in Louisiana and around the world, creating new opportunities for renewable energy, energy storage, smart grids, and electric vehicles. By embracing these technologies and innovations, Louisiana can reduce its dependence on fossil fuels, lower its carbon footprint, and build a more sustainable energy system for the future.
Review Questions
- What are the primary fossil fuel sources in the U.S., and how has their share in the energy mix changed over the past few decades?
- What are the major renewable energy sources in the U.S., and how have their contributions evolved recently?
- What is the role of nuclear energy in the U.S. energy landscape, and what are the benefits and concerns?
- How do fossil fuels, especially oil and natural gas, shape Louisiana’s energy landscape and economy?
- What is the renewable energy potential in Louisiana, and how viable are sources like solar, wind, and biomass for adoption?
- What policies or initiatives are driving renewable energy development and integration in Louisiana?
Critical Thinking Questions
- How do the primary energy sources in the U.S. affect progress toward sustainable energy goals?
- What strategies can ensure a secure and sustainable transition from non-renewable to renewable energy?
- How does dependence on specific energy sources impact the U.S. economy, and what risks does it pose?
- How can emerging technologies revolutionize the energy sector to optimize resources and reduce environmental impact?
- What are the economic and environmental trade-offs of Louisiana’s reliance on oil and gas, and how can the state balance energy needs with sustainability?
- How can renewable energy integration improve Louisiana’s resilience to climate change and extreme weather?
Key Terms
- Coal formation – formed from the dead remains of plant matter buried in dirt over millions of years and exposed to heat and pressure.
- Decarbonization – minimizing carbon usage or shifting from fossil fuels to low or zero-carbon energy sources.
- Emissions – release of chemical pollutants that may cause environmental harm.
- Energy efficiency – reducing the amount of consumed energy to complete tasks.
- Gas production – refers to the processing and manufacturing of natural gases.
- Geothermal energy – uses heat from the Earth’s internal geologic processes to produce electricity or provide heating.
- Natural gas – formed from the dead remains of plants and fossilized remains of animals buried in dirt over millions of years and exposed to heat and pressure.
- Nuclear energy – release of energy from nuclear fission reactions. This energy is captured to generate electricity.
- Oil formation – formed from the dead remains of plants and fossilized remains of animals buried in dirt over millions of years and exposed to heat and pressure.
- Solar energy – capturing energy from the sun to passively or actively generate electricity.
- Wind energy – converts kinetic energy into electrical energy by using moving air to spin turbines for power generation.
References and Additional Reading
Renewable Energy in the United States:
Davis, S. J., & Socolow, R. H. (2014). “Commitment accounting of CO2 emissions.” Environmental Research Letters, 9(8), 084018.
Gillingham, K., Rapson, D., & Wagner, G. (2016). “The rebound effect and energy efficiency policy.” Review of Environmental Economics and Policy, 10(1), 68–88.
Jacobson, M. Z., Delucchi, M. A., Bauer, Z. A., Goodman, S. C., Chapman, W. E., Cameron, M. A., … & Yip, F. S. (2015). “100% clean and renewable wind, water, and sunlight (WWS) all-sector energy roadmaps for the 50 United States.” Energy & Environmental Science, 8(7), 2093–2117.
Kroposki, B., Johnson, B., Zhang, Y., Gevorgian, V., Denholm, P., Hodge, B. M., … & Cahan, D. (2017). “Achieving a 100% renewable grid: Operating electric power systems with extremely high levels of variable renewable energy.” IEEE Power and Energy Magazine, 15(2), 61–73.
Sovacool, B. K. (2016). “How Long Will It Take? Conceptualizing the Temporal Dynamics of Energy Transitions.” Energy Research & Social Science, 13, 202–215.
Non-renewable Energy in the United States:
Brandt, A. R., Heath, G. A., Kort, E. A., O’Sullivan, F., Pétron, G., Jordaan, S. M., … & Wilcox, J. (2014). “Methane leaks from North American natural gas systems.” Science, 343(6172), 733–735.
Davis, S. J., Caldeira, K., & Matthews, H. D. (2010). “Future CO2 emissions and climate change from existing energy infrastructure.” Science, 329(5997), 1330–1333.
Hughes, J. D. (2013). “Energy: A reality check on the shale revolution.” Nature, 494(7437), 307–308.
Mach, K. J., & Plantinga, A. J. (2007). “The influence of prices and policies on the demand for natural gas: Evidence from the residential market in the United States.” Resource and Energy Economics, 29(1), 15–37.
Rogner, H. H. (1997). “An assessment of world hydrocarbon resources.” Annual Review of Energy and the Environment, 22(1), 217–262.
Renewable Energy in Louisiana:
Dusseault, D. A., & Jackson, S. R. (2015). “Onshore Energy in Louisiana.” Gulf Coast Association of Geological Societies Transactions, 65, 143–147.
Lehman, L. (2014). “Hydropower in Louisiana: A Renewable Energy Opportunity.” Louisiana State University Digital Commons, LSU Master’s Theses, 4011.
Mercer, B. M., & Johnson, W. (2018). “Analysis of Biomass Resources in Louisiana and Their Potential for Renewable Energy Production.” International Journal of Renewable Energy Development, 7(1), 11–21.
Miller, K., & Williams, J. H. (2017). “State of Solar: Louisiana.” National Renewable Energy Laboratory (NREL), NREL/FS-7A40-68870.
Mullen, M. (2019). “Wind Energy in Louisiana: Analysis and Potential.” Louisiana State University Digital Commons, LSU Master’s Theses, 4952.
Non-renewable Energy in Louisiana:
Center for Energy Studies, Louisiana State University. (2020). “Louisiana Petrochemical Industry Economic Impact Analysis.”
Cunningham, R. (2016). “The Economic Impact of the Oil and Gas Industry in Louisiana.” Louisiana State University Digital Commons, LSU Doctoral Dissertations, 2653.
Kirkpatrick, D. W., & Scott, B. J. (2016). “Offshore Oil and Gas in Louisiana: Past, Present, and Prospects for the Future.” Gulf Coast Association of Geological Societies Transactions, 66, 261–274.
Louisiana Department of Natural Resources. (2021). “Louisiana’s Oil and Natural Gas Industry: Economic Impact Report.”
Louisiana Oil & Gas Association. (2019). “Louisiana Oil & Gas Industry Economic Impact Report.”
Media Attributions
- River Bend Nuclear Station, Unit 1, in St. Francisville, Louisiana © Nuclear Regulatory Commission is licensed under a CC BY (Attribution) license
- Oil and Gas Formation © U.S. Energy Information Administration is licensed under a Public Domain license
- Coal formation © U.S. Energy Information Administration is licensed under a Public Domain license
- U.S. Primary Energy Consumption by Energy Source, 2022 © U.S. Energy Information Administration
- 1200px-Garzweiler.strip.mine is licensed under a CC BY-SA (Attribution ShareAlike) license
- Mountaintop remove in Eunice, West Virginia is licensed under a Public Domain license
- Mercury Food Chain © Bretwood Higman is licensed under a CC BY (Attribution) license
- 1200px-West_Texas_Pumpjack is licensed under a Public Domain license
- Oil_Platform_Crew_Transfer is licensed under a Public Domain license
- 1200px-Schematic_cross-section_of_general_types_of_oil_and_gas_resources_and_the_orientations_of_production_wells_used_in_hydraulic_fracturing is licensed under a Public Domain license
- Figure 4.10 Natural Gas EIA Monthly Review Report © U.S. Energy Information Administration is licensed under a Public Domain license
- Total all sectors energy source by month © U.S. Energy Information Administration is licensed under a Public Domain license
- Nuclear_fission_chain_reaction.svg © MikeRun is licensed under a CC BY-SA (Attribution ShareAlike) license
- Solar energy sun and panel © NASA Solar Dynamics Observatory
- Roscoe_Wind_Farm_in_West_Texas © Matthew T. Rader is licensed under a CC BY-SA (Attribution ShareAlike) license
- 900px-Diagram-VaporDominatedGeothermal © Wendell A. Duffield and John H. Sass is licensed under a Public Domain license