7 Ionic and Molecular Compounds and Bonding
Chapter Learning Objectives
After successful completion of the assignments in this section, you will be able to demonstrate competency in the following areas:
- Define the components and types of ionic compounds (CLO6)
- Model electron configuration by using Lewis structures (CLO6)(CLO7)
- Apply the octet rule for Lewis structures (CLO6)(CLO7)
- Discuss everyday situations related to molecular structures (CLO8)
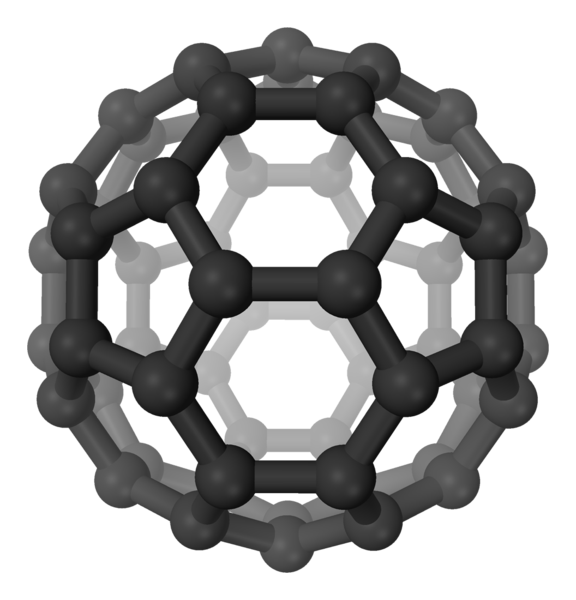
Ionic and Molecular Compounds
In ordinary chemical reactions, the nucleus of each atom (and thus the identity of the element) remains unchanged. Electrons, however, can be added to atoms by transfer from other atoms, lost by transfer to other atoms, or shared with other atoms. The transfer and sharing of electrons among atoms govern the chemistry of the elements. During the formation of some compounds, atoms gain or lose electrons and form electrically charged particles called ions.
As you have learned, ions are atoms or molecules bearing an electrical charge. An atom that gains one or more electrons will exhibit a negative charge and is called an anion. Positively charged atoms called cations are formed when an atom loses one or more electrons. You can use the periodic table to predict whether an atom will form an anion or a cation, and you can often predict the charge of the resulting ion. Atoms of many main-group metals lose enough electrons to leave them with the same number of electrons as an atom of the preceding noble gas.
Ionic Compounds
When an element composed of atoms that readily lose electrons (a metal) reacts with an element composed of atoms that readily gain electrons (a nonmetal), a transfer of electrons usually occurs, producing ions. The compound formed by this transfer is stabilized by the electrostatic attractions (ionic bonds) between the ions of opposite charge present in the compound.
A compound that contains ions and is held together by ionic bonds is called an ionic compound (or salts). The periodic table can help us recognize many of the compounds that are ionic: When a metal is combined with one or more nonmetals, the compound is usually ionic. This guideline works well for predicting ionic compound formation for most of the compounds typically encountered in an introductory chemistry course. However, it is not always true (for example, aluminum chloride, AlCl3, is not ionic).
In every ionic compound, the total number of positive charges of the cations equals the total number of negative charges of the anions. Thus, ionic compounds are electrically neutral overall, even though they contain positive and negative ions. We can use this observation to help us write the formula of an ionic compound. The formula of an ionic compound must have a ratio of ions such that the numbers of positive and negative charges are equal.
The properties of ionic compounds shed some light on the nature of ionic bonds. Ionic solids exhibit a crystalline structure and tend to be rigid and brittle; they also tend to have high melting and boiling points, which suggests that ionic bonds are very strong. Ionic solids are also poor conductors of electricity for the same reason—because electricity is the movement of electrons, the strength of ionic bonds prevents ions from moving freely in the solid state. Most ionic solids, however, dissolve readily in water. Once dissolved or melted, ionic compounds are excellent conductors of electricity and heat because the ions can move about freely.
Molecular Compounds
Many compounds do not contain ions but instead consist solely of discrete, neutral molecules. These molecular compounds (covalent compounds) result when atoms share, rather than transfer (gain or lose), electrons. We can often identify molecular compounds on the basis of their physical properties. Under normal conditions, molecular compounds often exist as gases, low-boiling liquids, and low-melting solids, although many important exceptions exist.
Whereas ionic compounds are usually formed when a metal and a nonmetal combine, covalent compounds are usually formed by a combination of nonmetals. Thus, the periodic table can help us recognize many of the compounds that are covalent. While we can use the positions of a compound’s elements in the periodic table to predict whether it is ionic or covalent at this point in our study of chemistry, you should be aware that this is a very simplistic approach that does not account for a number of interesting exceptions. Shades of gray exist between ionic and molecular compounds, and you’ll learn more about those later.
Neutral atoms and their associated ions have very different physical and chemical properties. Sodium atoms form sodium metal, a soft, silvery-white metal that burns vigorously in air and reacts explosively with water. Chlorine atoms form chlorine gas, Cl2, a yellow-green gas that is extremely corrosive to most metals and very poisonous to animals and plants. The vigorous reaction between the elements sodium and chlorine forms the white, crystalline compound sodium chloride, common table salt, which contains sodium cations and chloride anions (Figure 7.2). The compound composed of these ions exhibits properties entirely different from the properties of the elements sodium and chlorine. Chlorine is poisonous, but sodium chloride is essential to life; sodium atoms react vigorously with water, but sodium chloride simply dissolves in water.
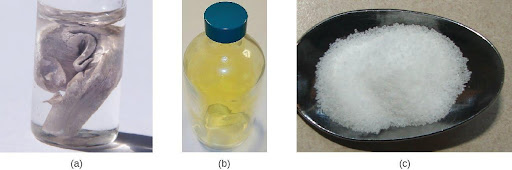
The Formation of Ionic Compounds
Binary ionic compounds are composed of just two elements: a metal (which forms the cations) and a nonmetal (which forms the anions). For example, NaCl is a binary ionic compound. We can think about the formation of such compounds in terms of the periodic properties of the elements. Many metallic elements have relatively low ionization potentials (the energy necessary to remove an electron from an atom) and lose electrons easily. These elements lie to the left in a period or near the bottom of a group on the periodic table. Nonmetal atoms have relatively high electron affinities and thus readily gain electrons lost by metal atoms, thereby filling their valence shells. Nonmetallic elements are found in the upper-right corner of the periodic table.
As all substances must be electrically neutral, the total number of positive charges on the cations of an ionic compound must equal the total number of negative charges on its anions.
It is important to note that the formula for an ionic compound does not represent the physical arrangement of its ions. It is incorrect to refer to a sodium chloride (NaCl) “molecule” because there is not a single ionic bond, per se, between any specific pair of sodium and chloride ions. The attractive forces between ions are isotropic—the same in all directions—meaning that any particular ion is equally attracted to all of the nearby ions of opposite charge. This results in the ions arranging themselves into a tightly bound, three-dimensional lattice structure. Sodium chloride, for example, consists of a regular arrangement of equal numbers of Na+ cations and Cl− anions (Figure 7.3).
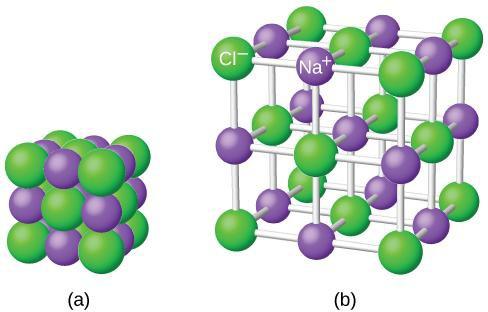
The strong electrostatic attraction between Na+ and Cl− ions holds them tightly together in solid NaCl. It requires 769 kJ of energy to dissociate one mole of solid NaCl into separate gaseous Na+ and Cl− ions.
Covalent Bonds
Ionic bonding results from the electrostatic attraction of oppositely charged ions that are typically produced by the transfer of electrons between metallic and nonmetallic atoms. Covalent bonding results from the mutual attraction of atoms for a “shared” pair of electrons and are typically formed between two nonmetals. Covalent bonds are formed between two atoms when both have similar tendencies to attract electrons to themselves (i.e., when both atoms have identical or fairly similar ionization energies and electron affinities). For example, two hydrogen atoms bond covalently to form an H2 molecule; each hydrogen atom in the H2 molecule has two electrons stabilizing it, giving each atom the same number of valence electrons (two) as the noble gas He.
Compounds that contain covalent bonds exhibit different physical properties than ionic compounds. Because the attraction between molecules, which are electrically neutral, is weaker than that between electrically charged ions, covalent compounds generally have much lower melting and boiling points than ionic compounds. In fact, many covalent compounds are liquids or gases at room temperature, and in their solid states they are typically much softer than ionic solids. Furthermore, whereas ionic compounds are good conductors of electricity when dissolved in water, most covalent compounds are insoluble in water; since they are electrically neutral, they are poor conductors of electricity in any state.
Pure vs. Polar Covalent Bonds
If the atoms that form a covalent bond are identical, as in H2, Cl2, and other diatomic molecules, then the electrons in the bond must be shared equally. We refer to this as a pure covalent bond. Electrons shared in pure covalent bonds have an equal probability of being near each nucleus.
In the case of Cl2, each atom starts off with seven valence electrons, and each Cl shares one electron with the other, forming one covalent bond: Cl+Cl⟶Cl2
(See Lewis structure diagram for Cl2 below.)
The total number of electrons around each individual atom consists of six nonbonding electrons and two shared (i.e., bonding) electrons for eight total electrons, matching the number of valence electrons in the noble gas argon. Since the bonding atoms are identical, Cl2 also features a pure covalent bond.
When the atoms linked by a covalent bond are different, the bonding electrons are shared, but no longer equally. Instead, the bonding electrons are more attracted to one atom than the other, giving rise to a shift of electron density toward that atom. This unequal distribution of electrons is known as a polar covalent bond, characterized by a partial positive charge on one atom and a partial negative charge on the other. The atom that attracts the electrons more strongly acquires the partial negative charge and vice versa. For example, the electrons in the H–Cl bond of a hydrogen chloride molecule spend more time near the chlorine atom than near the hydrogen atom. Thus, in an HCl molecule, the chlorine atom carries a partial negative charge and the hydrogen atom has a partial positive charge. Figure 7.4 shows the distribution of electrons in the H–Cl bond. Note that the shaded area around Cl is much larger than it is around H, compared to the even distribution of electrons in the H2 nonpolar bond.
We sometimes designate the positive and negative atoms in a polar covalent bond using a lowercase Greek letter “delta,” δ, with a plus sign or minus sign to indicate whether the atom has a partial positive charge (δ+) or a partial negative charge (δ–). This symbolism is shown for the H–Cl molecule in Figure 7.4.
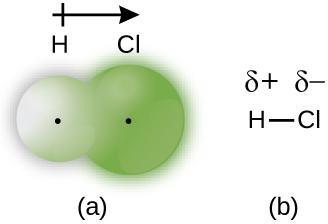
Metallic Bonds
When metal atoms bond in metallic bonds their valence electrons are shared among many atoms. These valence electrons can move around and are not fixed to any particular atom or pair of atoms, unlike both ionic and covalent bonds. Metals have unique properties like their conductivity and malleability because these electrons shared between the metal atoms can move easily. Atoms in metals are arranged in a crystalline structure that is held together by the strong bonds between the cations and the free “sea” of electrons.
Electronegativity
Whether a bond is nonpolar or polar covalent is determined by a property of the bonding atoms called electronegativity. Electronegativity is a measure of the tendency of an atom to attract electrons (or electron density) toward itself. It determines how the shared electrons are distributed between the two atoms in a bond. The more strongly an atom attracts the electrons in its bonds, the larger its electronegativity. Electrons in a polar covalent bond are shifted toward the more electronegative atom; thus, the more electronegative atom is the one with the partial negative charge. The greater the difference in electronegativity, the more polarized the electron distribution and the larger the partial charges of the atoms.
Figure 7.5 shows the electronegativity values of the elements as proposed by one of the most famous chemists of the twentieth century: Linus Pauling. In general, electronegativity increases from left to right across a period in the periodic table and decreases down a group. The nonmetals, which lie in the upper right, tend to have the highest electronegativities, with fluorine as the most electronegative element of all (EN = 4.0). Metals tend to be less electronegative elements, and the group 1 metals have the lowest electronegativities. Note that noble gases are excluded from this figure because these atoms usually do not share electrons with other atoms since they have a full valence shell. (While noble gas compounds such as XeO2 do exist, they can only be formed under extreme conditions and thus they do not fit neatly into the general model of electronegativity.)
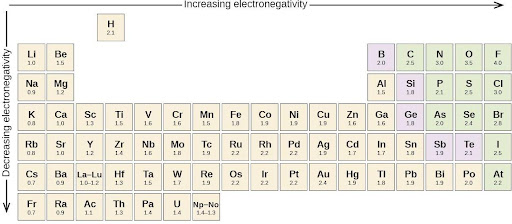
Lewis Symbols
We use Lewis symbols to describe valence electron configurations of atoms and monatomic ions. A Lewis symbol consists of an elemental symbol surrounded by one dot for each of its valence electrons:
Lewis Structures
We also use Lewis symbols to indicate the formation of covalent bonds, which are shown in Lewis structures, drawings that describe the bonding in molecules and polyatomic ions. For example, when two chlorine atoms form a chlorine molecule, they share one pair of electrons:
The Lewis structure indicates that each Cl atom has three pairs of electrons that are not used in bonding (called lone pairs) and one shared pair of electrons (written between the atoms). A dash (or line) is sometimes used to indicate a shared pair of electrons:
A single shared pair of electrons is called a single bond. Each Cl atom interacts with eight valence electrons: the six in the lone pairs and the two in the single bond.
The Octet Rule
The other halogen molecules (F2, Br2, I2, and At2) form bonds like those in the chlorine molecule: one single bond between atoms and three lone pairs of electrons per atom. This allows each halogen atom to have a noble gas electron configuration. The tendency of main-group atoms to form enough bonds to obtain eight valence electrons is known as the octet rule.
The number of bonds that an atom can form can often be predicted from the number of electrons needed to reach an octet (eight valence electrons); this is especially true of the nonmetals of the second period of the periodic table (C, N, O, and F). For example, each atom of a group 14 element has four electrons in its outermost shell and therefore requires four more electrons to reach an octet. These four electrons can be gained by forming four covalent bonds, as illustrated here for carbon in CCl4 (carbon tetrachloride) and silicon in SiH4 (silane). Because hydrogen only needs two electrons to fill its valence shell, it is an exception to the octet rule. The transition elements and inner transition elements also do not follow the octet rule:
Group 15 elements such as nitrogen have five valence electrons in the atomic Lewis symbol: one lone pair and three unpaired electrons. To obtain an octet, these atoms form three covalent bonds, as in NH3 (ammonia). Oxygen and other atoms in group 16 obtain an octet by forming two covalent bonds:
Double and Triple Bonds
As previously mentioned, when a pair of atoms shares one pair of electrons, we call this a single bond. However, a pair of atoms may need to share more than one pair of electrons in order to achieve the necessary octet. A double bond forms when two pairs of electrons are shared between a pair of atoms, as between the carbon and oxygen atoms in CH2O (formaldehyde) and between the two carbon atoms in C2H4 (ethylene):
A triple bond forms when three electron pairs are shared by a pair of atoms, as in carbon monoxide (CO) and the cyanide ion (CN−):
Writing Lewis Structures with the Octet Rule
For very simple molecules and molecular ions, we can write the Lewis structures by merely pairing up the unpaired electrons on the constituent atoms. See these examples:
Example 7.1
WRITING LEWIS STRUCTURES
NASA’s Cassini-Huygens mission detected a large cloud of toxic hydrogen cyanide (HCN) on Titan, one of Saturn’s moons. Titan also contains ethane (H3CCH3), acetylene (HCCH), and ammonia (NH3). What are the Lewis structures of these molecules?
Solution
Check Your Learning: Both carbon monoxide, CO, and carbon dioxide, CO2, are products of the combustion of fossil fuels. Both of these gases also cause problems: CO is toxic and CO2 has been implicated in global climate change. What are the Lewis structures of these two molecules?
ANSWER:
Check Your Understanding
The content in this chapter is adapted from multiple sources. The overall chapter structure and the section on the nature of science is adapted from Exploring the Physical World and used under a Creative Commons Attribution-NonCommercial-ShareAlike 4.0 International License. The sections on the formation of ionic and molecular compounds, covalent bonds, electronegativity, and Lewis symbols and structures are adapted from Chemistry 2e by OpenStax and are used under a Creative Commons-Attribution 4.0 International License. Other content and interactive activities are the original work of the authors of this text and are shared under a Creative Commons-Attribution 4.0 International License.
Media Attributions
- Fig 7.1 © Benjah-bmm27 is licensed under a Public Domain license
- Fig 7.2 © OpenStax is licensed under a CC BY (Attribution) license
- Fig. 7.3 © OpenStax is licensed under a CC BY (Attribution) license
- Fig. 7.4 © OpenStax is licensed under a CC BY (Attribution) license
- Fig. 7.5 © OpenStax is licensed under a CC BY (Attribution) license
pure substances that are comprised of two or more elements
atom in which the number of subatomic particles is not equal
negative ion formed by the loss of electrons
positive ion formed by the loss of electrons
table of the chemical elements arranged in order of atomic number, usually in rows, so that elements with similar atomic structure (and hence similar chemical properties) appear in vertical columns
electrostatic forces of attraction between oppositely charged cations and anions
compound that contains ions and is held together by ionic bonds
two or more atoms joined by strong forces called chemical bonds
compounds that result when atoms share rather than transfer electrons; also called covalent compounds
qualities and characteristics of individual substances that describe and identify them
ionic compounds composed of just two elements, a metal which forms the cations and a nonmetal which forms the anions
chemical bonds that involve the sharing of electrons to form electron pairs between atoms
covalent bonds in which electrons are shared equally between atoms within the compound
bond between different atoms in which electrons are not shared equally
bonds that result from the electrostatic attraction between metal cations and delocalized electrons
outer electrons of an atom that have the highest energy and are more easily lost or shared than the core electrons
measure of the tendency of an atom to attract electrons
elemental symbol surrounded by one dot for each of its valence electrons
drawings that describe the bonding in molecules and polyatomic ions
tendency of main-group atoms to form enough bonds to obtain eight valence electrons is known as the octet rule
when a pair of atoms shares one pair of electrons
when two pairs of electrons are shared between a pair of atoms
when three electron pairs are shared by a pair of atoms