8 Matter and Energy
Transformation and Conservation
Learning Objectives
After successful completion of this section, you will be able to demonstrate competency in the following areas:
- Explain and apply the law of conservation of energy (CLO1)(CLO2)(CLO3)(CLO5)(CLO6)
- Define energy, kinetic energy, and gravitational potential energy (CLO2)(CLO3)
- Analyze situations that involve kinetic and potential energy (CLO2)(CLO3)
- Calculate kinetic and potential energies (CLO2)(CLO5)
- Discuss the conservation of mass (CLO1)(CLO6)
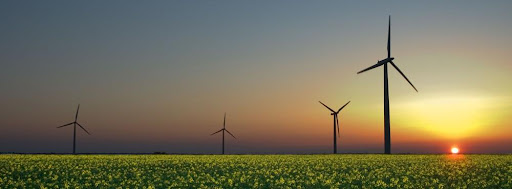
Introduction to Energy
Energy plays an essential role both in everyday events and in scientific phenomena. You can no doubt name many forms of energy, from that provided by our foods, to the energy we use to run our cars, to the sunlight that warms us on the beach. You can also cite examples of what people call energy that may not be scientific, such as someone having an energetic personality. Energy not only has many interesting forms; it is involved in almost all phenomena and is one of the most important concepts of physics. What makes it even more important is that the total amount of energy in the universe is constant. Energy can change forms, but it cannot appear from nothing or disappear without a trace. Energy is thus one of a handful of physical quantities that we say is conserved.
Conservation of energy (as physicists like to call the principle that energy can neither be created nor destroyed) is based on experiment. Even as scientists discovered new forms of energy, conservation of energy has always been found to apply. Perhaps the most dramatic example of this was supplied by Einstein when he suggested that mass is equivalent to energy (his famous equation E=mc2).
From a societal viewpoint, energy is one of the major building blocks of modern civilization. Energy resources are key limiting factors to economic growth. The world use of energy resources, especially oil, continues to grow, with ominous consequences economically, socially, politically, and environmentally.
There is no simple and accurate scientific definition for energy. Energy is characterized by its many forms and the fact that it is conserved. We can loosely define energy as the ability to do work, admitting that in some circumstances not all energy is available to do work. Work is intimately related to energy and how energy moves from one system to another or changes form.
Chemical Changes to Matter
Matter undergoes two types of changes: physical changes which do not change the composition of the original substance, only its appearance and other physical attributes, and chemical changes which result in the formation of a new substance with different chemical and physical properties from the original substance.
Chemical changes and their accompanying changes in energy are important parts of our everyday world. The macronutrients in food (proteins, fats, and carbohydrates) undergo metabolic reactions that provide the energy to keep our bodies functioning. We burn a variety of fuels (gasoline, natural gas, coal) to produce energy for transportation, heating, and the generation of electricity. Industrial chemical reactions use enormous amounts of energy to produce raw materials (such as iron and aluminum). Energy is then used to manufacture those raw materials into useful products, such as cars, skyscrapers, and bridges.
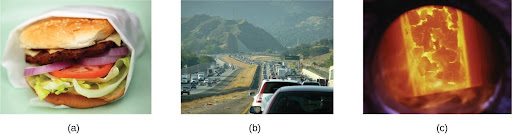
Over 90% of the energy we use comes originally from the sun in the form of radiant energy. Every day, the sun provides the earth with almost 10,000 times the amount of energy necessary to meet all of the world’s energy needs for that day. Our challenge is to find ways to convert and store incoming solar energy so that it can be used in reactions or chemical processes that are both convenient and non-polluting. Plants and many bacteria capture solar energy through photosynthesis. We release the energy stored in plants when we burn wood or plant products such as ethanol. We also use this energy to fuel our bodies by eating food that comes directly from plants or from animals that got their energy by eating plants. Burning coal and petroleum also releases stored solar energy: These fuels are fossilized plant and animal matter.
Forms of Energy
Energy can be defined as the capacity to supply heat or do work. One type of work (w) is the process of causing matter to move against an opposing force. For example, we do work when we inflate a bicycle tire—we move matter (the air in the pump) against the opposing force of the air already in the tire.
Like matter, energy comes in different types. One scheme classifies energy into two types; potential and kinetic energy. Potential energy is the energy an object has because of its relative position, composition, or condition. Kinetic energy is the energy that an object possesses because of its motion. Water at the top of a waterfall or dam has potential energy because of its position; when it flows downward through generators, it has kinetic energy that can be used to do work and produce electricity in a hydroelectric plant. A battery has potential energy because the chemicals within it can produce electricity that can do work.
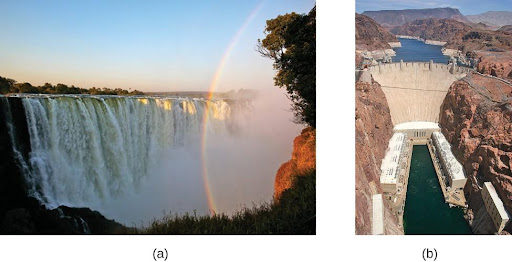
The energy associated with translational motion (kinetic energy) is expressed KE = (1/2) mv2. Kinetic energy is a form of energy associated with the motion of a particle, single body, or system of objects moving together. The unit of measure for kinetic energy is the joule. We are aware that it takes energy to get an object, like a car, up to speed, but it may be a bit surprising that kinetic energy is proportional to velocity squared. This proportionality means, for example, that a car traveling at 100 km/h has four times the kinetic energy it has at 50 km/h, helping to explain why high-speed collisions are so devastating. We will now consider an example to illustrate the relationship between work and energy.
Example 8.1
Suppose a 30.0-kg package on a roller belt conveyor system is moving at 0.500 m/s. What is its kinetic energy?
- Strategy: Because the mass (m) and speed or velocity (v) are given, the kinetic energy can be calculated from its definition as given in the equation KE = (1/2) mv2.
- Solution: The kinetic energy is given by KE = (1/2) mv2. Entering known values gives KE = 0.5 * (30.0kg)*(0.500m/s)2 which yields KE=3.75kg⋅m2/s2=3.75 J.
- Discussion: Note that the unit of kinetic energy is the joule (kg⋅m2/s2). It is interesting that, although this is a fairly massive package, its kinetic energy is not large at this relatively low speed. This fact is consistent with the observation that people can move packages like this without exhausting themselves.
In Figure 8.4, if the object is lifted straight up we refer to the energy the object has due to gravity as potential energy (PE) gained by the object, recognizing that this is energy stored in the gravitational field of Earth.
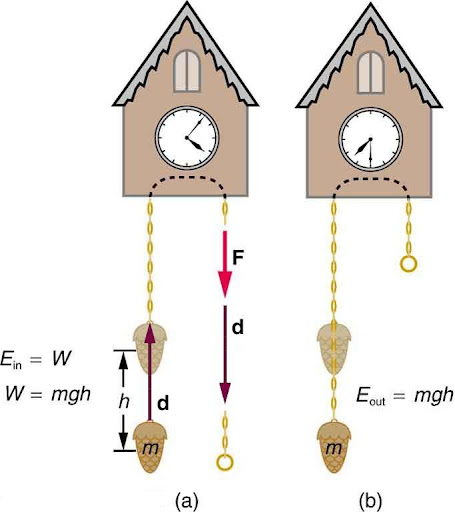
(b) As the weight moves downward, this gravitational potential energy is transferred to the cuckoo clock.
Why do we use the word “system” when discussing potential energy? Potential energy is a property of a system rather than of a single object—due to its physical position. An object’s gravitational potential energy is due to its position relative to the surroundings within the Earth-object system. The force applied to the object is an external force, from outside the system. When it does positive work it increases the gravitational potential energy of the system. Because gravitational potential energy depends on relative position, we need a reference level at which to set the potential energy equal to 0. We usually choose this point to be Earth’s surface, but this point is arbitrary; what is important is the difference in gravitational potential energy, because this difference is what relates to the work done. The difference in gravitational potential energy of an object (in the Earth-object system) between two rungs of a ladder will be the same for the first two rungs as for the last two rungs.
Converting between Potential Energy and Kinetic Energy
Gravitational potential energy may be converted to other forms of energy, such as kinetic energy. If we release the mass, gravitational force will do an amount of work equal to the mgh on it, thereby increasing its kinetic energy by that same amount (i.e., converting E to KE).
More precisely, we define the change in gravitational potential energy ΔPE to be ΔPE = mgh. You may also see the change in gravitational potential energy written as ΔUg=mgy. These two equations both represent the potential energy due to gravity and can be used interchangeably. In these equations m represents the mass of the object and g represents the gravitational acceleration (9.81m/s2). Both y and h represent the change in height, but y more specifically refers to the change in distance in the y direction.
For example, if a 0.500-kg mass hung from a cuckoo clock is raised 1.00 m, then its change in gravitational potential energy is ΔPE = mgh = (0.500 kg)(9.80m/s2)(1.00 m) = 4.90 kg⋅m2/s2 = 4.90 J.
Mechanical Energy
Mechanical energy is kinetic energy and any potential energy related to the potential for an object to move. We deal with all other forms of energy by lumping them into a single group called other energy (OE). Then we can state the conservation of energy in equation form as
KEi + PEi + OEi = KEf + PEf + OEf
where subscript i stands for initial, and subscript f stands for final.
All types of energy and work can be included in this very general statement of conservation of energy. Kinetic energy is KE, mechanical potential energy is represented by PE, and all other energies are included as OE. This equation applies to all previous examples; in those situations OE was constant, and so it subtracted out and was not directly considered.
Calculating Potential and Kinetic Energy
To calculate the potential energy of an object, we use the following formula:
Potential Energy (PE) = mass times the acceleration due to gravity times height
PE = mgh = N*h (g= 9.8 m/s2)
1 Newton (N) = 1kg*1m/s2 or 1kg.m/s2
Where:
PE is potential energy in N
m is the mass in kg
g is the acceleration due to gravity in m/s2
h is the height of the object in m
To calculate the kinetic energy in a system, we use this formula:
Kinetic energy (KE) = ½ mass times velocity squared
KE = ½ mv2
Where:
KE is kinetic energy in N
m is the mass in kg
v2 is the velocity of the object in m/s squared
Conservation of Energy & Matter
When one substance is converted into another, there is always an associated conversion of one form of energy into another. Heat is usually released or absorbed, but sometimes the conversion involves light, electrical energy, or some other form of energy. For example, chemical energy (a type of potential energy) is stored in the molecules that compose gasoline. When gasoline is combusted within the cylinders of a car’s engine, the rapidly expanding gaseous products of this chemical reaction generate mechanical energy (a type of kinetic energy) when they move the cylinders’ pistons. Other forms of energy include sound energy, nuclear energy, magnetic energy, gravitational energy, radiant energy, and thermal energy.
Energy, as we have noted, is conserved, making it one of the most important physical quantities in nature. The law of conservation of energy can be stated as follows:
Total energy is constant in any process. It may change in form or be transferred from one system to another, but the total remains the same.
Making Connections: Usefulness of the Energy Conservation Principle
The fact that energy is conserved and has many forms makes it very important. You will find that energy is discussed in many contexts, because it is involved in all processes. It will also become apparent that many situations are best understood in terms of energy and that problems are often most easily conceptualized and solved by considering energy.
When does OE play a role? One example occurs when a person eats. Food is oxidized with the release of carbon dioxide, water, and energy. Some of this chemical energy is converted to kinetic energy when the person moves, to potential energy when the person changes altitude, and to thermal energy OE.
Problem-Solving Strategies for Energy
You will find the following problem-solving strategies useful whenever you deal with energy. The strategies help in organizing and reinforcing energy concepts. In fact, they are used in the examples presented in this chapter. The familiar general problem-solving strategies presented earlier—involving identifying physical principles, knowns, unknowns, checking units, and so on—continue to be relevant here.
Step 1. Determine the system of interest, identify what information is given and what quantity is to be calculated. A sketch will help.
Step 2. Examine all the forces involved and determine whether you know or are given the potential energy from the work done by the forces. Then use step 3 or step 4.
Step 3. If you know that you can apply conservation of mechanical energy simply in terms of potential and kinetic energy, the equation expressing conservation of energy is KEi + PEi = KEf + PEf.
Step 4. You have already identified the types of energy involved (in step 2). Before solving for the unknown, eliminate terms wherever possible to simplify the algebra. For example, choose h = 0 at either the initial or the final point, so the gravitational potential energy (PEg) is zero there. Then solve for the unknown in the customary manner.
Step 5. Check the answer to see if it is reasonable. Once you have solved a problem, reexamine the forms of work and energy to see if you have set up the conservation of energy equation correctly. For example, work done against friction should be negative, potential energy at the bottom of a hill should be less than that at the top, and so on. Also check to see that the numerical value obtained is reasonable. For example, the final speed of a skateboarder who coasts down a 3-m-high ramp could reasonably be 20 km/h, but not 80 km/h.
Transformation of Energy
The transformation of energy from one form into others is happening all the time. The chemical energy in food is converted into thermal energy through metabolism; light energy is converted into chemical energy through photosynthesis. In a larger example, the chemical energy contained in coal is converted into thermal energy as it burns to turn water into steam in a boiler. This thermal energy in the steam in turn is converted to mechanical energy as it spins a turbine, which is connected to a generator to produce electrical energy. (In all of these examples, not all of the initial energy is converted into the forms mentioned.)
Another example of energy conversion occurs in a solar cell. Sunlight impinging on a solar cell (see Figure 8.5) produces electricity, which in turn can be used to run an electric motor. Energy is converted from the primary source of solar energy into electrical energy and then into mechanical energy.
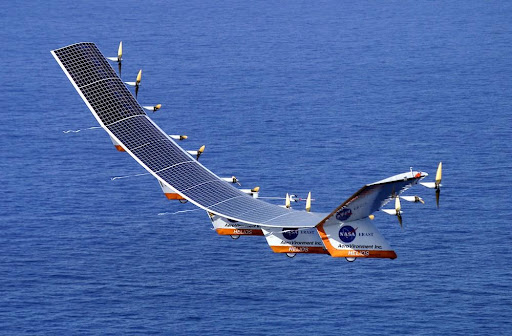
Conservation of Mass
The mass of an object is a measure of the amount of matter in it. One way to measure an object’s mass is to measure the force it takes to accelerate the object. It takes much more force to accelerate a car than a bicycle because the car has much more mass. A more common way to determine the mass of an object is to use a balance to compare its mass with a standard mass.
Although weight is related to mass, it is not the same thing. Weight refers to the force that gravity exerts on an object. This force is directly proportional to the mass of the object. The weight of an object changes as the force of gravity changes, but its mass does not. An astronaut’s mass does not change just because she goes to the moon. But her weight on the moon is only one-sixth her earth-bound weight because the moon’s gravity is only one-sixth that of the earth’s. She may feel “weightless” during her trip when she experiences negligible external forces (gravitational or any other), although she is, of course, never “massless.”
The law of conservation of matter summarizes many scientific observations about matter. It states:
There is no detectable change in the total quantity of matter present when matter converts from one type to another (a chemical change) or changes among solid, liquid, or gaseous states (a physical change).
Brewing beer and the operation of batteries provide examples of the conservation of matter. During the brewing of beer, the ingredients (water, yeast, grains, malt, hops, and sugar) are converted into beer (water, alcohol, carbonation, and flavoring substances) with no actual loss of substance. This is most clearly seen during the bottling process, when glucose turns into ethanol and carbon dioxide, and the total mass of the substances does not change. This can also be seen in a lead-acid car battery: The original substances (lead, lead oxide, and sulfuric acid), which are capable of producing electricity, are changed into other substances (lead sulfate and water) that do not produce electricity, with no change in the actual amount of matter.
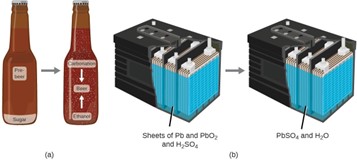
Although this conservation law holds true for all conversions of matter, convincing examples are few and far between because, outside of the controlled conditions in a laboratory, we seldom collect all of the material that is produced during a particular conversion. For example, when you eat, digest, and assimilate food, all of the matter in the original food is preserved. But because some of the matter is incorporated into your body, and much is excreted as various types of waste, it is challenging to verify by measurement.
Check Your Understanding
The content in this chapter is adapted from multiple sources. The overall chapter structure is adapted from Exploring the Physical World and used under a Creative Commons Attribution-NonCommercial-ShareAlike 4.0 International License. The section on chemical changes to matter is adapted from Chemistry 2e by OpenStax and is used under a Creative Commons-Attribution 4.0 International License. The sections on potential and kinetic energy, mechanical energy, and conservation of energy and matter are adapted from College Physics 2e by OpenStax and are used under a Creative Commons-Attribution 4.0 International License. Other content and interactive activities are the original work of the authors of this text and are shared under a Creative Commons-Attribution 4.0 International License.
Media Attributions
- Fig. 8.1 © Jürgen from Sandesneben, Germany is licensed under a CC BY (Attribution) license
- Fig. 8.2 © OpenStax is licensed under a CC BY (Attribution) license
- Fig. 8.3 © OpenStax is licensed under a CC BY (Attribution) license
- Fig. 8.4 © OpenStax is licensed under a CC BY (Attribution) license
- Fig. 8.5 © NASA is licensed under a Public Domain license
- Fig. 8.6 © OpenStax is licensed under a CC BY (Attribution) license
capacity of a physical system to perform work
force causing the movement—or displacement—of an object
process in which one or more substances are altered into one or more new and different substances with different physical and chemical properties
energy emitted by electromagnetic waves that travel through space without any medium
energy that is stored in an object due to its position relative to some zero position
energy of motion
The SI derived unit used to measure energy or work. One joule is equal to the energy used to accelerate a body with a mass of one kilogram using one newton of force over a distance of one meter
energy from the movement of atoms or molecules. It may be considered as energy relating to temperature. Also known as thermal energy
energy (both kinetic and potential) in the charged particles of an atom that can be used to apply force and/or do work
energy that is produced by breaking or forming chemical bonds between atoms and molecules
sum of the kinetic and potential energy of a body
form of kinetic energy caused by the physical vibration of air particles or molecules
form of energy released from the nucleus, the core of atoms, made up of protons and neutrons
energy that results from a magnetic field
potential energy associated with gravitational force
energy from the movement of atoms or molecules. It may be considered as energy relating to temperature; also referred to as heat
total energy of a system remains constant, though energy may transform into another form
a dimensionless quantity representing the amount of matter in a particle or object
the force acting on an object due to gravity
in a closed or isolated system, matter cannot be created or destroyed. It can change forms but is conserved