4 Properties of Materials, Chemical Reactions, and Heat
Learning Objectives
After successful completion of the assignments in this section, you will be able to demonstrate competency in the following areas:
- Identify the states of matter (CLO2)
- Write and balance chemical equations (CLO7)
- Define three common types of chemical reactions (precipitation, acid-base, and oxidation-reduction) (CLO6)
- Classify chemical reactions as one of these three types given appropriate descriptions or chemical equations (CLO6)(CLO7)
- Identify common acids and bases (CLO6)(CLO8)
- Define and distinguish between heat and temperature (CLO2)(CLO3)
- Express temperature using Fahrenheit, Celsius, and Kelvin scales (CLO2)(CLO5)
- Discuss heat transfer applications and relate them to phase changes of matter (CLO2)(CLO3)
- Define methods of heat transfer: conduction, convection, and radiation (CLO2)(CLO3)
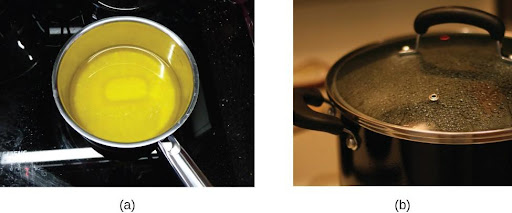
Properties of Materials
The characteristics that distinguish one substance from another are called properties. A physical property is a characteristic of matter that is not associated with a change in its chemical composition. Familiar examples of physical properties include density, color, hardness, melting and boiling points, and electrical conductivity. Some physical properties, such as density and color, may be observed without changing the physical state of the matter. Other physical properties, such as the melting temperature of iron or the freezing temperature of water, can only be observed as matter undergoes a physical change.
States of Matter
Solids, liquids, and gases are the three states of matter commonly found on Earth (Figure 4.2). A solid is rigid and possesses a definite shape. A liquid flows and takes the shape of its container, except that it forms a flat or slightly curved upper surface when acted upon by gravity. (In zero gravity, liquids assume a spherical shape.) Both liquid and solid samples have volumes that are very nearly independent of pressure. A gas takes both the shape and volume of its container.
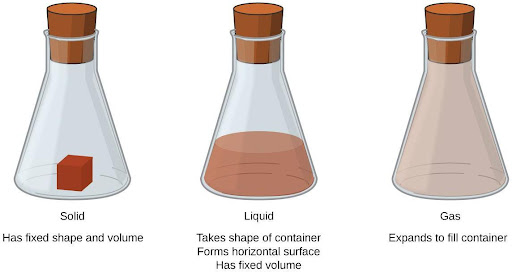
A fourth state of matter, plasma, occurs naturally in the interiors of stars. Plasma is a gaseous state of matter that contains appreciable numbers of electrically charged particles (Figure 4.3). The presence of these charged particles imparts unique properties to plasmas that justify their classification as a state of matter distinct from gases. In addition to stars, plasmas are found in some other high-temperature environments (both natural and man-made), such as lightning strikes, certain television screens, and specialized analytical instruments used to detect trace amounts of metals.
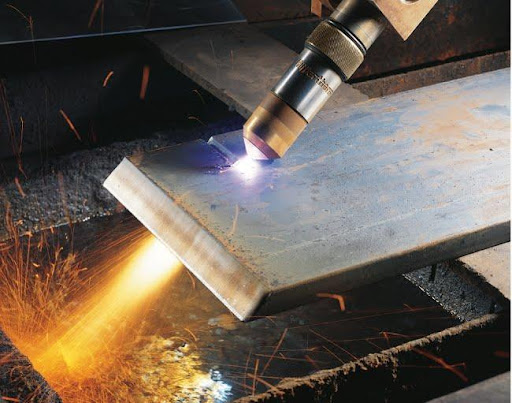
Some samples of matter appear to have properties of solids, liquids, and/or gases at the same time. This can occur when the sample is composed of many small pieces. For example, we can pour sand as if it were a liquid because it is composed of many small grains of solid sand. Matter can also have properties of more than one state when it is a mixture, such as with clouds. Clouds appear to behave somewhat like gases, but they are actually mixtures of air (gas) and tiny particles of water (liquid or solid).
Classification of Matter
Matter can be classified into several categories. Two broad categories are mixtures and pure substances. A pure substance has a constant composition. All specimens of a pure substance have exactly the same makeup and properties. Any sample of sucrose (table sugar) consists of 42.1% carbon, 6.5% hydrogen, and 51.4% oxygen by mass. Any sample of sucrose also has the same physical properties, such as melting point, color, and sweetness, regardless of the source from which it is isolated.
Pure substances may be divided into two classes: elements and compounds. Pure substances that cannot be broken down into simpler substances by chemical changes are called elements. Iron, silver, gold, aluminum, sulfur, oxygen, and copper are familiar examples of the more than 100 known elements, of which about 90 occur naturally on the earth, and two dozen or so have been created in laboratories.
Pure substances that are comprised of two or more elements are called compounds. Compounds may be broken down by chemical changes to yield either elements or other compounds, or both.
The properties of combined elements are different from those in the free, or uncombined, state. For example, white crystalline sugar (sucrose) is a compound resulting from the chemical combination of the element carbon, which is a black solid in one of its uncombined forms, and the two elements hydrogen and oxygen, which are colorless gases when uncombined. Free sodium (an element that is a soft, shiny, metallic solid) and free chlorine (an element that is a yellow-green gas) combine to form sodium chloride (table salt), a compound that is a white, crystalline solid.
A mixture is composed of two or more types of matter that can be present in varying amounts and can be separated by physical changes, such as evaporation (you will learn more about this later). A mixture with a composition that varies from point to point is called a heterogeneous mixture. Italian dressing is an example of a heterogeneous mixture. Its composition can vary because it may be prepared from varying amounts of oil, vinegar, and herbs. It is not the same from point to point throughout the mixture—one drop may be mostly vinegar, whereas a different drop may be mostly oil or herbs because the oil and vinegar separate and the herbs settle. Other examples of heterogeneous mixtures are chocolate chip cookies (we can see the separate bits of chocolate, nuts, and cookie dough) and granite (we can see the quartz, mica, feldspar, and more).
A homogeneous mixture, also called a solution, exhibits a uniform composition and appears visually the same throughout. An example of a solution is a sports drink, consisting of water, sugar, coloring, flavoring, and electrolytes mixed together uniformly. Each drop of a sports drink tastes the same because each drop contains the same amounts of water, sugar, and other components. Note that the composition of a sports drink can vary—it could be made with somewhat more or less sugar, flavoring, or other components, and still be a sports drink. Other examples of homogeneous mixtures include air, maple syrup, gasoline, and a solution of salt in water.
Although there are just over 100 elements, tens of millions of chemical compounds result from different combinations of these elements. Each compound has a specific composition and possesses definite chemical and physical properties that distinguish it from all other compounds. And, of course, there are innumerable ways to combine elements and compounds to form different mixtures.
Atoms and Molecules
An atom is the smallest particle of an element that has the properties of that element and can enter into a chemical combination. Consider the element gold, for example. Imagine cutting a gold nugget in half, then cutting one of the halves in half, and repeating this process until a piece of gold remained that was so small that it could not be cut in half (regardless of how tiny your knife may be). This minimally sized piece of gold is an atom (from the Greek atomos, meaning “indivisible”). Such an atom would no longer be gold if it were divided further.
An atom is so small that its size is difficult to imagine. One of the smallest things we can see with our unaided eye is a single thread of a spider web: These strands are about 1/10,000 of a centimeter (0.0001 cm) in diameter. Although the cross-section of one strand is almost impossible to see without a microscope, it is huge on an atomic scale. A single carbon atom in the web has a diameter of about 0.000000015 centimeter, and it would take about 7,000 carbon atoms to span the diameter of the strand. An atom is so light that its mass is also difficult to imagine. A billion lead atoms (1,000,000,000 atoms) weigh about 3 × 10−13 grams, a mass that is far too light to be weighed on even the world’s most sensitive balances. It would require over 300,000,000,000,000 lead atoms (300 trillion, or 3 × 1014) to be weighed, and they would weigh only 0.0000001 gram.
It is rare to find collections of individual atoms. Only a few elements—such as the gases helium, neon, and argon—consist of a collection of individual atoms that move about independently of one another. Other elements—such as the gases hydrogen, nitrogen, oxygen, and chlorine—are composed of units that consist of pairs of atoms. One form of the element phosphorus consists of units composed of four phosphorus atoms. The element sulfur exists in various forms, one of which consists of units composed of eight sulfur atoms. These units are called molecules. A molecule consists of two or more atoms joined by strong forces called chemical bonds. The atoms in a molecule move around as a unit, much like the cans of soda in a six-pack or a bunch of keys joined together on a single key ring. A molecule may consist of two or more identical atoms, as in the molecules found in the elements hydrogen, oxygen, and sulfur, or it may consist of two or more different atoms, as in the molecules found in water. Each water molecule is a unit that contains 2 hydrogen atoms and 1 oxygen atom. Each glucose molecule is a unit that contains 6 carbon atoms, 12 hydrogen atoms, and 6 oxygen atoms. Like atoms, molecules are incredibly small and light. If an ordinary glass of water were enlarged to the size of the earth, the water molecules inside it would be about the size of golf balls.
Physical and Chemical Changes
A physical change is a change in the state or properties of matter without any accompanying change in the chemical identities of the substances contained in the matter. Physical changes are observed when wax melts, when sugar dissolves in coffee, and when steam condenses into liquid water (Figure 4.1). Other examples of physical changes include magnetizing and demagnetizing metals (as is done with common anti-theft security tags) and grinding solids into powders (which can sometimes yield noticeable changes in color). In each of these examples, there is a change in the physical state, form, or properties of the substance, but no change in its chemical composition.
The change of one type of matter into another type (or the inability to change) is a chemical property. Examples of chemical properties include flammability, toxicity, acidity, and many other types of reactivity. Iron, for example, combines with oxygen in the presence of water to form rust; chromium does not oxidize (Figure 4.4). Nitroglycerin is very dangerous because it explodes easily; neon poses almost no hazard because it is very unreactive.
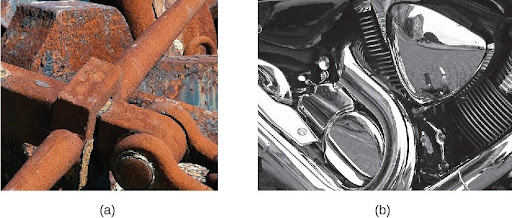
A chemical change always produces one or more types of matter that differ from the matter present before the change. The formation of rust is a chemical change because rust is a different kind of matter than the iron, oxygen, and water present before the rust formed. The explosion of nitroglycerin is a chemical change because the gases produced are very different kinds of matter from the original substance. Other examples of chemical changes include reactions that are performed in a lab (such as copper reacting with nitric acid), all forms of combustion (burning), and food being cooked, digested, or rotting (Figure 4.5).
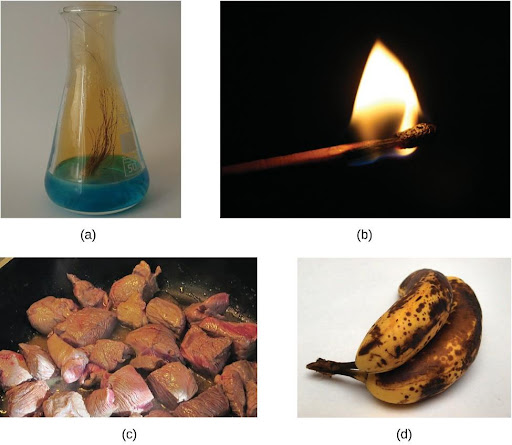
Balancing Equations
When atoms gain or lose electrons to yield ions, or combine with other atoms to form molecules, their symbols are modified or combined to generate chemical formulas that appropriately represent these species. Extending this symbolism to represent both the identities and the relative quantities of substances undergoing a chemical (or physical) change involves writing and balancing a chemical equation. Consider as an example the reaction between one methane molecule (CH4) and two diatomic oxygen molecules (O2) to produce one carbon dioxide molecule (CO2) and two water molecules (H2O).
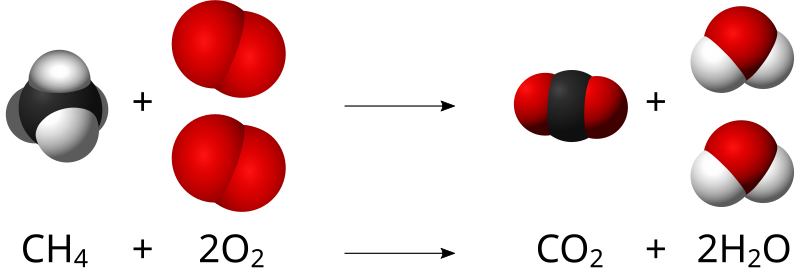
The chemical equation in Figure 4.6 is balanced, meaning that equal numbers of atoms for each element involved in the reaction are represented on the reactant (left) and product (right) sides. This is a requirement the equation must satisfy to be consistent with the law of conservation of matter. It may be confirmed by simply summing the numbers of atoms on either side of the arrow and comparing these sums to ensure they are equal. Note that the number of atoms for a given element is calculated by multiplying the coefficient of any formula containing that element by the element’s subscript in the formula. If an element appears in more than one formula on a given side of the equation, the number of atoms represented in each must be computed and then added together. For example, both product species in the example reaction, CO2 and H2O, contain the element oxygen, and so the number of oxygen atoms on the product side of the equation is
(1 CO2 molecule × 2 O atoms per CO2 molecule) + (2 H2O molecules × 1 O atom per H2O molecule) = 4 O atoms
The equation for the reaction between methane and oxygen to yield carbon dioxide and water is confirmed to be balanced per this approach, as shown here:
CH4 + 2O2⟶CO2 + 2H2O
Element | Reactants | Products | Balanced? |
C | 1 × 1 = 1 | 1 × 1 = 1 | 1 = 1, yes |
H | 4 × 1 = 4 | 2 × 2 = 4 | 4 = 4, yes |
O | 2 × 2 = 4 | (1 × 2) + (2 × 1) = 4 | 4 = 4, yes |
A balanced chemical equation often may be derived from a qualitative description of some chemical reaction by a fairly simple approach known as balancing by inspection. Consider as an example the decomposition of water to yield molecular hydrogen and oxygen. This process is represented qualitatively by an unbalanced chemical equation:
H2O⟶H2 + O2 (unbalanced)
Comparing the number of H and O atoms on either side of this equation confirms its imbalance:
Element | Reactants | Products | Balanced? |
H | 1 × 2 = 2 | 1 × 2 = 2 | 2 = 2, yes |
O | 1 × 1 = 1 | 1 × 2 = 2 | 1 ≠ 2, no |
The numbers of H atoms on the reactant and product sides of the equation are equal, but the numbers of O atoms are not. To achieve balance, the coefficients of the equation may be changed as needed. Note that the coefficients must be whole numbers and cannot be fractions or decimals. Keep in mind, of course, that the formula subscripts define, in part, the identity of the substance, and so these cannot be changed without altering the qualitative meaning of the equation. For example, changing the reactant formula from H2O to H2O2 would yield balance in the number of atoms, but doing so also changes the reactant’s identity (it’s now hydrogen peroxide and not water). The O atom balance may be achieved by changing the coefficient for H2O to 2.
2H2O⟶H2 + O2 (unbalanced)
Element | Reactants | Products | Balanced? |
H | 2 × 2 = 4 | 1 × 2 = 2 | 4 ≠ 2, no |
O | 2 × 1 = 2 | 1 × 2 = 2 | 2 = 2, yes |
The H atom balance was upset by this change, but it is easily reestablished by changing the coefficient for the H2 product to 2.
2H2O⟶2H2 + O2 (balanced)
Element | Reactants | Products | Balanced? |
H | 2 × 2 = 4 | 2 × 2 = 4 | 4 = 4, yes |
O | 2 × 1 = 2 | 1 × 2 = 2 | 2 = 2, yes |
These coefficients yield equal numbers of both H and O atoms on the reactant and product sides, and the balanced equation is, therefore:
2H2O⟶2H2 + O2
Example 4.1
Write a balanced equation for the decomposition of ammonium nitrate to form molecular nitrogen, molecular oxygen, and water. (Hint: Balance oxygen last, since it is present in more than one molecule on the right side of the equation.)
ANSWER: 2NH4NO3⟶2N2 + O2 + 4H2O
Types of Chemical Reactions
Humans interact with one another in various and complex ways, and we classify these interactions according to common patterns of behavior. When two humans exchange information, we say they are communicating. When they hit each other with their fists, we say they are fighting. Faced with a wide range of varied interactions between chemical substances, scientists have likewise found it convenient (or even necessary) to classify chemical interactions by identifying common patterns of reactivity. This section will provide an introduction to three of the most prevalent types of chemical reactions: precipitation, acid-base, and oxidation-reduction.
Precipitation Reactions
A precipitation reaction is one in which dissolved substances react to form one (or more) solid products. Many reactions of this type involve the exchange of ions between ionic compounds in aqueous solution and are sometimes referred to as double displacement, double replacement, or metathesis reactions. These reactions are common in nature and are responsible for the formation of coral reefs in ocean waters and kidney stones in animals. They are used widely in industry for the production of a number of commodity and specialty chemicals.
The extent to which a substance may be dissolved in water, or any solvent, is quantitatively expressed as its solubility, defined as the maximum concentration of a substance that can be achieved under specified conditions. Substances with relatively large solubilities are said to be soluble. A substance will precipitate when solution conditions are such that its concentration exceeds its solubility. Substances with relatively low solubilities are said to be insoluble, and these are the substances that readily precipitate from solution.
Lead iodide is a bright yellow solid that was formerly used as an artist’s pigment known as iodine yellow (Figure 4.6). The properties of pure PbI2 crystals make them useful for fabrication of X-ray and gamma ray detectors.
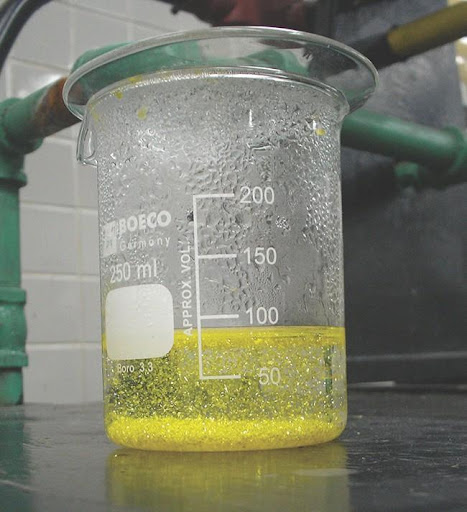
Acid-Base Reactions
An acid-base reaction is one in which a hydrogen ion, H+, is transferred from one chemical species to another. Such reactions are of central importance to numerous natural and technological processes, ranging from the chemical transformations that take place within cells and the lakes and oceans, to the industrial-scale production of fertilizers, pharmaceuticals, and other substances essential to society.
For the purposes of this brief introduction, we will consider only the more common types of acid-base reactions that take place in aqueous solutions. In this context, an acid is a substance that will dissolve in water to yield hydronium ions, H3O+. As an example, consider the equation shown here:
HCl(aq) + H2O(aq)⟶Cl−(aq) + H3O+(aq)
The process represented by this equation confirms that hydrogen chloride is an acid. When dissolved in water, H3O+ ions are produced by a chemical reaction in which H+ ions are transferred from HCl molecules to H2O molecules (Figure 4.7).
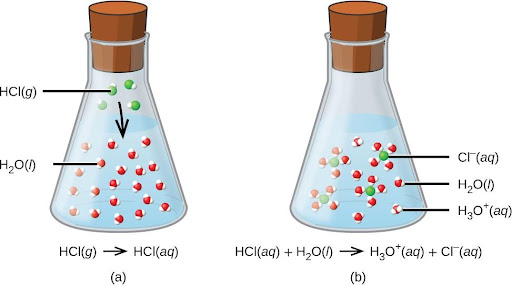
The nature of HCl is such that its reaction with water as just described is essentially 100% efficient: Virtually every HCl molecule that dissolves in water will undergo this reaction. Acids that completely react in this fashion are called strong acids, and HCl is one among just a handful of common acid compounds that are classified as strong. A far greater number of compounds behave as weak acids and only partially react with water, leaving a large majority of dissolved molecules in their original form and generating a relatively small amount of hydronium ions. Weak acids are commonly encountered in nature, being the substances partly responsible for the tangy taste of citrus fruits, the stinging sensation of insect bites, and the unpleasant smells associated with body odor. A familiar example of a weak acid is acetic acid, the main ingredient in food vinegars (Figure 4.8).
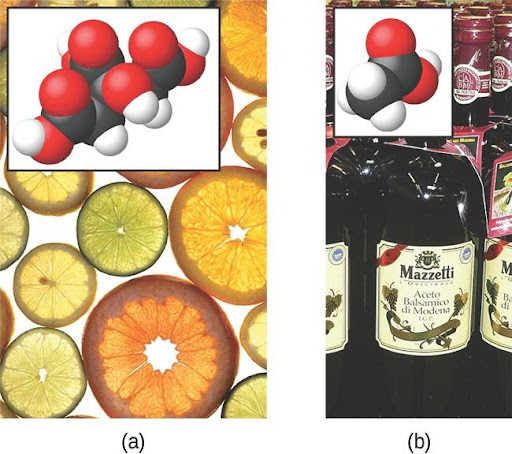
A base is a substance that will dissolve in water to yield hydroxide ions, OH−. The most common bases are ionic compounds composed of alkali or alkaline earth metal cations (groups 1 and 2) combined with the hydroxide ion—for example, NaOH and Ca(OH)2. Unlike the acid compounds discussed previously, these compounds do not react chemically with water; instead they dissolve and dissociate, releasing hydroxide ions directly into the solution. For example, KOH and Ba(OH)2 dissolve in water and dissociate completely to produce cations (K+ and Ba2+, respectively) and hydroxide ions (OH−). These bases, along with other hydroxides that completely dissociate in water, are considered strong bases.
Consider as an example the dissolution of lye (sodium hydroxide) in water:
NaOH⟶Na+ + OH−
This equation confirms that sodium hydroxide is a base. When dissolved in water, NaOH dissociates to yield Na+ and OH− ions. This is also true for any other ionic compound containing hydroxide ions. Since the dissociation process is essentially complete when ionic compounds dissolve in water under typical conditions, NaOH and other ionic hydroxides are all classified as strong bases.
Chemistry in Everyday Life: Stomach Antacids
Our stomachs contain a solution of roughly 0.03 M HCl (molarity), which helps us digest the food we eat. The burning sensation associated with heartburn is a result of the acid of the stomach leaking through the muscular valve at the top of the stomach into the lower reaches of the esophagus. The lining of the esophagus is not protected from the corrosive effects of stomach acid the way the lining of the stomach is, and the results can be very painful. When we have heartburn, it feels better if we reduce the excess acid in the esophagus by taking an antacid. As you may have guessed, antacids are bases. One of the most common antacids is calcium carbonate, CaCO3. The reaction: CaCO3 + 2HCl⇌CaCl2 + H2O + CO2 not only neutralizes stomach acid, it also produces CO2, which may result in a satisfying belch.
Many people like to put lemon juice or vinegar, both of which are acids, on cooked fish (Figure 4.9). It turns out that fish have volatile amines (bases) in their systems, which are neutralized by the acids to yield involatile ammonium salts. This reduces the odor of the fish, and also adds a “sour” taste that we seem to enjoy.
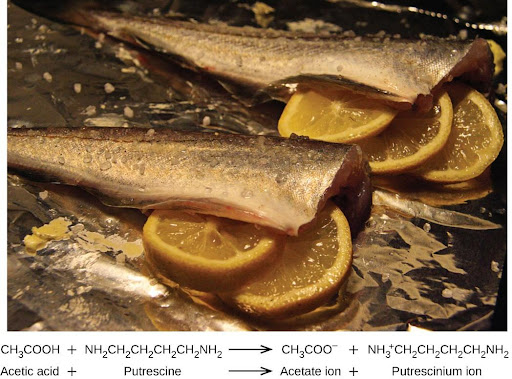
Pickling is a method used to preserve vegetables using a naturally produced acidic environment. The vegetable, such as a cucumber, is placed in a sealed jar submerged in a brine solution. The brine solution favors the growth of beneficial bacteria and suppresses the growth of harmful bacteria. The beneficial bacteria feed on starches in the cucumber and produce lactic acid as a waste product in a process called fermentation. The lactic acid eventually increases the acidity of the brine to a level that kills any harmful bacteria, which require a basic environment. Without the harmful bacteria consuming the cucumbers, they are able to last much longer than if they were unprotected. A byproduct of the pickling process changes the flavor of the vegetables with the acid making them taste sour.
Chemistry in Everyday Life: Culinary Chemistry
Examples of acid-base chemistry are abundant in the culinary world. One example is the use of baking soda, or sodium bicarbonate, in baking. NaHCO3 is a base. When it reacts with an acid such as lemon juice, buttermilk, or sour cream in a batter, bubbles of carbon dioxide gas are formed from the decomposition of the resulting carbonic acid, and the batter “rises.” Baking powder is a combination of sodium bicarbonate and one or more acid salts that react when the two chemicals come in contact with water in the batter.
Oxidation-Reduction Reactions
Earth’s atmosphere contains about 20% molecular oxygen, O2, a chemically reactive gas that plays an essential role in the metabolism of aerobic organisms and in many environmental processes that shape the world. The term oxidation was originally used to describe chemical reactions involving O2, but its meaning has evolved to refer to a broad and important reaction class known as oxidation-reduction (redox) reactions. A few examples of such reactions will be used to develop a clear picture of this classification.
Some redox reactions involve the transfer of electrons between reactant species to yield ionic products, such as the reaction between sodium and chlorine to yield sodium chloride:
2Na + Cl2⟶2NaCl
For redox reactions of this sort, the loss and gain of electrons define the complementary processes that occur:
Oxidation = loss of electrons
Reduction = gain of electrons
In this reaction, then, sodium is oxidized and chlorine undergoes reduction. Viewed from a more active perspective, sodium functions as a reducing agent (reductant), since it provides electrons to (or reduces) chlorine. The chlorine functions as an oxidizing agent (oxidant), as it effectively removes electrons from (oxidizes) sodium.
Reducing agent = species that is oxidized
Oxidizing agent = species that is reduced
Thermal Energy, Temperature, and Heat
Thermal energy is kinetic energy associated with the random motion of atoms and molecules. Temperature is a quantitative measure of “hot” or “cold.” When the atoms and molecules in an object are moving or vibrating quickly, they have a higher average kinetic energy (KE), and we say that the object is “hot.” When the atoms and molecules are moving slowly, they have lower average KE, and we say that the object is “cold” (Figure 4.10). Assuming that no chemical reaction or phase change (such as melting or vaporizing) occurs, increasing the amount of thermal energy in a sample of matter will cause its temperature to increase. And assuming that no chemical reaction or phase change (such as condensation or freezing) occurs, decreasing the amount of thermal energy in a sample of matter will cause its temperature to decrease.
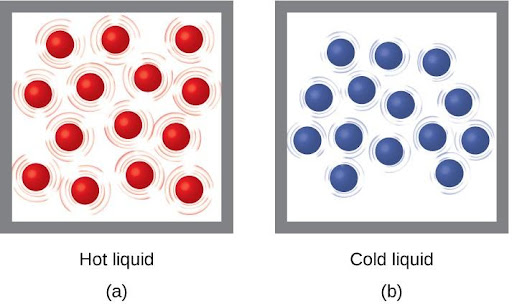
Most substances expand as their temperature increases and contract as their temperature decreases. This property can be used to measure temperature changes, as shown in Figure 4.11. The operation of many thermometers depends on the expansion and contraction of substances in response to temperature changes.
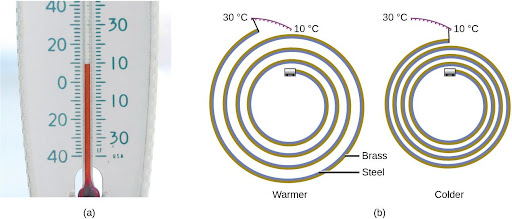
Heat Transfer: Conduction, Convection and Radiation
Heat transfer can happen three different ways; through conduction, convection and radiation. Conduction is heat transfer through contact between atoms. The conductivity of materials is influenced by their atomic structure and bonds. For example, metals are very conductive because they have free flowing electrons that can easily transfer thermal energy. Convection is heat transfer through the movement of gases or liquids. When these fluids are heated and move away from the heat source, they carry the thermal energy with them. A great example of convection is a space heater; the air is heated and then blown out of the heater, sending thermal energy with it out into the room and heating it up. Radiation is the heat transfer through electromagnetic waves. We experience heat from radiation every day from the sun, which transfers heat to the earth through solar radiation. Radiation is the only type of heat transfer that can travel through empty space, because both conduction and convection require a medium to transfer the thermal energy through.
Heat (q) is the transfer of thermal energy between two bodies at different temperatures. Heat flow (a redundant term, but one commonly used) increases the thermal energy of one body and decreases the thermal energy of the other. Suppose we initially have a high-temperature (and high-thermal-energy) substance, H, and a low temperature (and low thermal energy) substance, L. The atoms and molecules in H have a higher average KE than those in L. If we place substance H in contact with substance L, the thermal energy will flow spontaneously from substance H to substance L. The temperature of substance H will decrease, as will the average KE of its molecules; the temperature of substance L will increase, along with the average KE of its molecules. Heat flow will continue until the two substances are at the same temperature and therefore reach thermal equilibrium (Figure 4.12).
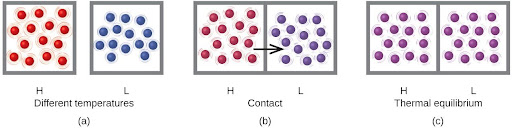
Matter undergoing chemical reactions and physical changes can release or absorb heat. A change that releases heat is called an exothermic process. For example, the combustion reaction that occurs when using an oxyacetylene torch is an exothermic process—this process also releases energy in the form of light as evidenced by the torch’s flame. A reaction or change that absorbs heat is an endothermic process. A cold pack used to treat muscle strains provides an example of an endothermic process. When the substances in the cold pack (water and a salt like ammonium nitrate) are brought together, the resulting process absorbs heat, leading to the sensation of cold.
Check Your Understanding
The content in this chapter is adapted from multiple sources. The overall chapter structure and the section on the nature of science is adapted from Exploring the Physical World and used under a Creative Commons Attribution-NonCommercial-ShareAlike 4.0 International License. The sections on properties of materials, physical and chemical changes, types of chemical reactions, and thermal energy are adapted from Chemistry 2e by OpenStax and is used under a Creative Commons-Attribution 4.0 International License. Other content and interactive activities are the original work of the authors of this text and are shared under a Creative Commons-Attribution 4.0 International License.
Media Attributions
- Fig. 4.1 © OpenStax is licensed under a CC BY (Attribution) license
- Fig 4.2 © OpenStax is licensed under a CC BY (Attribution) license
- Fig. 4.3 © Hypertherm is licensed under a CC0 (Creative Commons Zero) license
- Fig. 4.4 © OpenStax is licensed under a CC BY (Attribution) license
- Fig. 4.5 © OpenStax is licensed under a CC BY (Attribution) license
- Fig. 4.6 © Yulo1985 is licensed under a CC BY-SA (Attribution ShareAlike) license
- Fig. 4.7 © Der Kreole is licensed under a CC BY (Attribution) license
- Fig. 4.8 © OpenStax is licensed under a CC BY (Attribution) license
- Fig. 4.9 © OpenStax is licensed under a CC BY (Attribution) license
- Fig. 4.10 © OpenStax is licensed under a CC BY (Attribution) license
- Fig. 4.11 © OpenStax is licensed under a CC BY (Attribution) license
- Fig. 4.12 © OpenStax is licensed under a CC BY (Attribution) license
- Fig. 4.13 © OpenStax is licensed under a CC BY (Attribution) license
qualities and characteristics of individual substances that describe and identify them
characteristic of matter that is not associated with a change in its chemical composition
distinct forms in which matter can exist
universal force of attraction acting between all matter
substance for which all specimens have exactly the same makeup and properties
pure substances that cannot be broken down into simpler substances by chemical changes
pure substances that are comprised of two or more elements
substance composed of two or more types of matter that can be present in varying amounts and can be separated by physical changes
mixture with a composition that varies from point to point
a mixture that exhibits a uniform composition and appears visually the same throughout; also called a solution
a mixture that exhibits a uniform composition and appears visually the same throughout; also called a homogeneous mixture
smallest particle of an element that has the properties of that element and can enter into a chemical combination
two or more atoms joined by strong forces called chemical bonds
change in the state or properties of matter without any accompanying change in the chemical identities of the substances contained in the matter
process in which one or more substances are altered into one or more new and different substances with different physical and chemical properties
symbolic representations of chemical reactions in which the reactants and the products are expressed in terms of their respective chemical formulae
equation in which equal numbers of atoms for each element involved in the reaction are represented on the reactant (left) and product (right) sides
common patterns of reactivity between elements
reaction in which dissolved substances react to form one or more solid products
reaction in which a hydrogen ion, H+, is transferred from one chemical species to another
substance that will dissolve in water to yield hydronium ions, H3O+
substance that will dissolve in water to yield hydroxide ions, OH−
reactions that involves the transfer of electrons between chemical species; also known as oxidation-reduction reactions
process that occurs when an atom, molecule, or ion loses one or more electrons in a chemical reaction
process in which a chemical species decreases its oxidation number, usually by gaining electrons
energy from the movement of atoms or molecules. It may be considered as energy relating to temperature; also referred to as heat
energy of motion
a measure of the average kinetic energy of the particles in an object
heat transfer through contact between atoms
heat transfer through the movement of gases or liquids
heat transfer through electromagnetic waves
energy from the movement of atoms or molecules. It may be considered as energy relating to temperature. Also known as thermal energy
movement of thermal energy that increases the energy of one body and decreases the energy of the other body
change that releases heat