Chapter 10. The Skeletal System
Priscilla Stewart
Unit Outline
A. Bone Tissue and the Skeletal System
Part 1: The Functions of the Skeletal System
Part 4: Bone Formation and Development
- Cartilage Templates
- Intramembranous Ossification
- Endochondral Ossification
- How Bones Grow in Length
- How Bones Grow in Diameter
- Bone Remodeling
Part 2: The Appendicular Skeleton
Part 3: The Pelvic Girdle and Pelvis
Part 4: Bones of the Lower Limbs
Learning Objectives
At the end of this unit, you should be able to:
I. Describe the functions of the skeletal system and the five basic shapes of human bones.
II. Describe the structure and histology of the skeletal system.
III. Define and identify the following parts of a long bone: diaphysis, epiphysis, metaphysis, articular cartilage, periosteum, medullary cavity, and endosteum.
IV. Compare the composition and function of compact bone versus spongy bone.
V. Define ossification, compare intramembranous ossification with endochondral ossification, describe how a long bone grows in length and width, and specify how various factors might affect the rate of ossification and, by extension, the height of a mature individual.
VI. Describe four types of bone fractures.
VII. Specify the components of the axial and appendicular skeletons; describe the general function of each skeleton.
VIII. Describe the structure and function of a typical vertebra and explain how these differ in the case of the atlas and axis.
IX. Describe the components and functions of the pectoral girdle and the pelvic girdle.
X. Specify all bones and structures in the human skeleton covered in this unit.
XI. Describe the differences between the pelvis of a human female and that of a human male.
XII. Describe the major differences between the skeleton of an infant and that of an adult.
A. Bone Tissue and the Skeletal System
Your skeleton is a structure of living tissue that grows, repairs, and renews itself. The bones within it are dynamic and complex organs that serve a number of important functions, including some necessary to maintain homeostasis.
The skeletal system forms the rigid internal framework of the body. It consists of the bones, cartilages, and ligaments. Bones support the weight of the body, allow for body movements, and protect internal organs. Cartilage provides flexible strength and support for body structures such as the thoracic cage, the external ear, and the trachea and larynx. At joints of the body, cartilage can also unite adjacent bones or provide cushioning between them. Ligaments are the strong connective tissue bands that hold the bones at a moveable joint together and serve to prevent excessive movements of the joint that would result in injury. Providing movement of the skeleton are the muscles of the body, which are firmly attached to the skeleton via connective tissue structures called tendons. As muscles contract, they pull on the bones to produce movements of the body. Thus, without a skeleton, you would not be able to stand, run, or even feed yourself!
Each bone of the body serves a particular function, and therefore, bones vary in size, shape, and strength based on these functions. For example, the bones of the lower back and lower limb are thick and strong to support your body weight. Similarly, the size of a bony landmark that serves as a muscle attachment site on an individual bone is related to the strength of this muscle. Muscles can apply very strong pulling forces to the bones of the skeleton. To resist these forces, bones have enlarged bony landmarks at sites where powerful muscles attach. This means that not only the size of a bone, but also its shape, is related to its function. Bones are also dynamic organs that can modify their strength and thickness in response to changes in muscle strength or body weight. Thus, muscle attachment sites on bones will thicken if you begin a workout program that increases muscle strength. Similarly, the walls of weight-bearing bones will thicken if you gain body weight or begin pounding the pavement as part of a new running regimen. In contrast, a reduction in muscle strength or body weight will cause bones to become thinner. This may happen during a prolonged hospital stay, following limb immobilization in a cast, or going into the weightlessness of outer space. Even a change in diet, such as eating only soft food due to the loss of teeth, will result in a noticeable decrease in the size and thickness of the jaw bones.
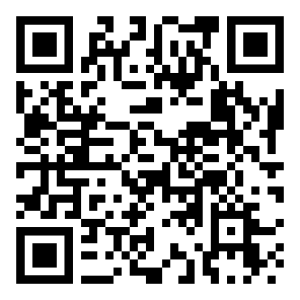
Part 1: The Functions of the Skeletal System
Bone, or osseous tissue, is a hard, dense connective tissue that forms most of the adult skeleton, the support structure of the body. In the areas of the skeleton where bones move (for example, the rib cage and joints), cartilage, a semi-rigid form of connective tissue, provides flexibility and smooth surfaces for movement. The skeletal system is the body system composed of bones and cartilage and performs the following critical functions for the human body:
- supports the body
- facilitates movement
- protects internal organs
- produces blood cells
- stores and releases minerals and fat
Support, Movement and Protection
The most apparent functions of the skeletal system are the gross functions—those visible by observation. Simply by looking at a person, you can see how the bones support, facilitate movement, and protect the human body.
Just as the steel beams of a building provide a scaffold to support its weight, the bones and cartilage of your skeletal system compose the scaffold that supports the rest of your body. Without the skeletal system, you would be a limp mass of organs, muscle, and skin.
Bones also facilitate movement by serving as points of attachment for your muscles. While some bones only serve as a support for the muscles, others also transmit the forces produced when your muscles contract. From a mechanical point of view, bones act as levers, and joints serve as fulcrums (Figure 10.1). Unless a muscle spans a joint and contracts, a bone is not going to move.
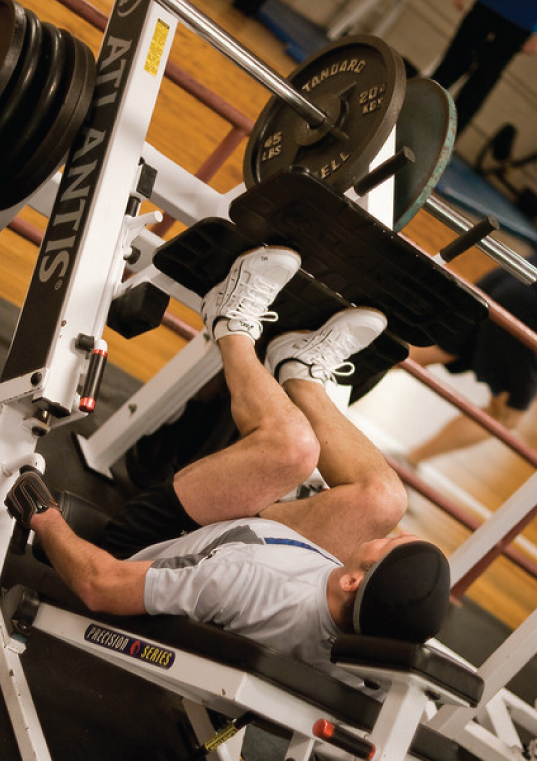

Bones also protect internal organs from injury by covering or surrounding them. For example, your ribs protect your lungs and heart; the bones of your vertebral column (spine) protect your spinal cord; and the bones of your cranium (skull) protect your brain (Figure 10.2).
Mineral Storage, Energy Storage, and Hematopoiesis
On a metabolic level, bone tissue performs several critical functions. For one, the bone matrix (ground substance) acts as a reservoir for a number of minerals important to the functioning of the body, especially calcium, and phosphorus. These minerals, incorporated into bone tissue, can be released back into the bloodstream to maintain levels needed to support physiological processes. Calcium ions, for example, are essential for muscle contractions and controlling the flow of other ions involved in the transmission of nerve impulses.
Bone also serves as a site for fat storage and blood cell production. The softer connective tissue that fills the interior of most bone is referred to as bone marrow (Figure 10.3). There are two types of bone marrow: yellow marrow and red marrow. Yellow marrow contains adipose tissue; the triglycerides stored in the adipocytes of the tissue can serve as a source of energy. Red marrow is where hematopoiesis—the production of blood cells—takes place. Red blood cells, white blood cells, and cell fragments called platelets are all produced in the red marrow.
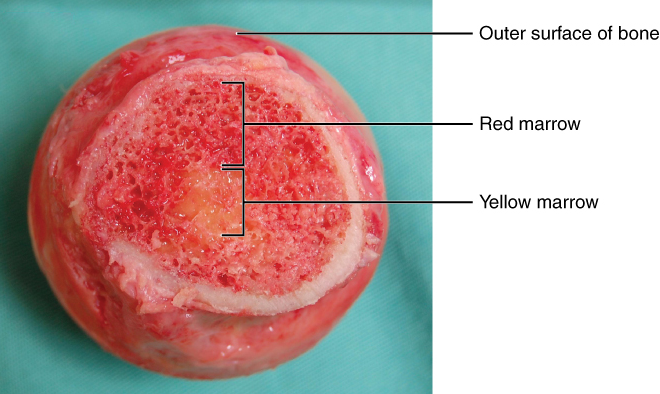
Test Your Knowledge
I. Specify the ways that the skeletal system functions in the human body.
II. Explain how the skeletal “protects” and “supports” the human body.
Part 2: Bone Classification
The 206 bones that compose the adult skeleton can be divided into five categories based on their shapes (Figure 10.4). Their shapes and their functions are related such that each categorical shape of bone has a distinct function.
Long Bones: A long bone is cylindrical in shape, with a diameter smaller than its height. Keep in mind, however, that the term describes the shape of a bone, not its size. Long bones are found in the arms (humerus, ulna, and radius) and legs (femur, tibia, and fibula), as well as in the fingers (metacarpals and phalanges) and toes (metatarsals and phalanges). Long bones function as levers; they move when muscles contract.
Short Bones: A short bone is one that is cube-like in shape, being approximately equal in length, width, and thickness. The only short bones in the human skeleton are in the carpals of the wrists and the tarsals of the ankles. Short bones provide stability and support as well as some limited motion.
Flat Bones: The term “flat bone” is somewhat of a misnomer because, although a flat bone is typically thin, it is also often curved. Examples include the cranial bones of the skull, the scapulae (shoulder blades), the sternum (breastbone), and the ribs. Flat bones serve as points of attachment for muscles and often protect internal organs.
Irregular Bones: An irregular bone is one that does not have any easily characterized shape and therefore does not fit any other classification. These bones tend to have more complex shapes, like the vertebrae that support the spinal cord and protect it from compressive forces. Many facial bones, particularly the ones containing sinuses, are classified as irregular bones.
Sesamoid Bones: A sesamoid bone is a small, round bone that, as the name suggests, is shaped like a sesame seed. These bones form in tendons (the sheaths of tissue that connect bones to muscles) where a great deal of pressure is generated in a joint. The sesamoid bones protect tendons by helping them overcome compressive forces. Sesamoid bones vary in number and placement from person to person but are typically found in tendons associated with the feet, hands, and knees. The patellae (singular = patella) are the only sesamoid bones found in common with every person.
Table 10.1 reviews bone classifications with their associated features, functions, and examples.
Bone classification | Features | Function(s) | Examples |
---|---|---|---|
Long | Cylinder-like shape, longer than it is wide | Leverage | Femur, tibia, fibula, metatarsals, humerus, ulna, radius, metacarpals, phalanges |
Short | Cube-like shape, approximately equal in length, width, and thickness | Provide stability & support while allowing for some motion | Carpals, tarsals |
Flat | Thin and curved | Points of attachment for muscles; protectors of internal organs | Sternum, ribs, scapulae, cranial bones |
Irregular | Complex shape | Protect internal organs | Vertebrae, facial bones |
Sesamoid | Small and round; embedded in tendons | Protect tendons from compressive forces | Patellae |
Test Your Knowledge
Part 3: Bone Structure
Bone tissue (osseous tissue) differs greatly from other tissues in the body. Bone is hard and many of its functions depend on that characteristic hardness. Later discussions in this chapter will show that bone is also dynamic in that its shape adjusts to accommodate stresses. This section will examine the gross anatomy of bone first and then move on to its histology.
Gross Anatomy of Bone
The structure of a long bone allows for the best visualization of all of the parts of a bone (Figure 10.5). A long bone has two parts: the diaphysis and the epiphysis. The diaphysis is the tubular shaft that runs between the proximal and distal ends of the bone. The hollow region in the diaphysis is called the medullary cavity, which is filled with yellow marrow. The walls of the diaphysis are composed of dense and hard compact bone. The wider section at each end of the bone is called the epiphysis (plural = epiphyses), which is filled with spongy bone. Red marrow fills the spaces in the spongy bone.
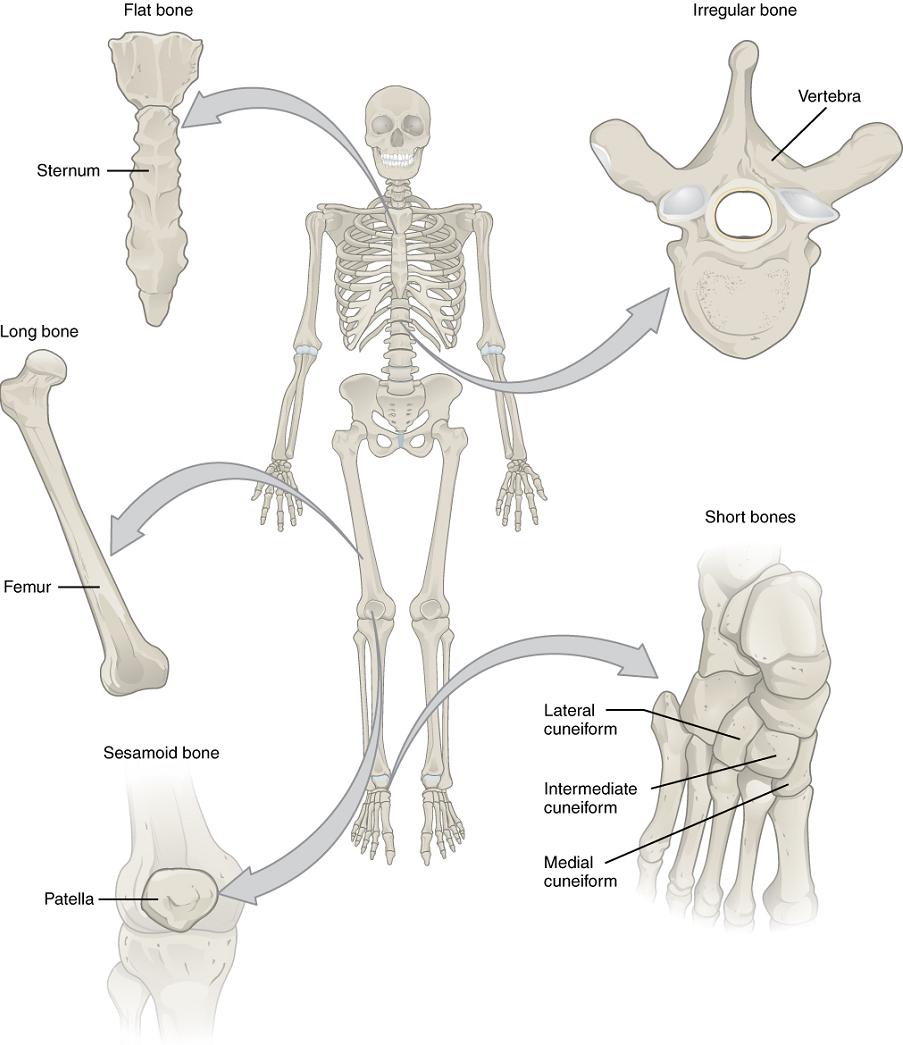
Each epiphysis meets the diaphysis at the metaphysis, the narrow area that contains the epiphyseal plate (growth plate), a layer of hyaline (transparent) cartilage in a growing bone. When the bone stops growing in early adulthood (approximately 18–21 years), the cartilage is replaced by osseous tissue and the epiphyseal plate becomes an epiphyseal line.
The medullary cavity has a delicate membranous lining called the endosteum (end- = “inside”; oste- = “bone”), where bone growth, repair, and remodeling occur. The outer surface of the bone is covered with a fibrous membrane called the periosteum (peri– = “around” or “surrounding”). The periosteum contains blood vessels, nerves, and lymphatic vessels that nourish compact bone. Tendons and ligaments also attach to bones at the periosteum. The periosteum covers the entire outer surface except where the epiphyses meet other bones to form joints (Figure 10.6). In this region, the epiphyses are covered with articular cartilage, a thin layer of cartilage that reduces friction and acts as a shock absorber.
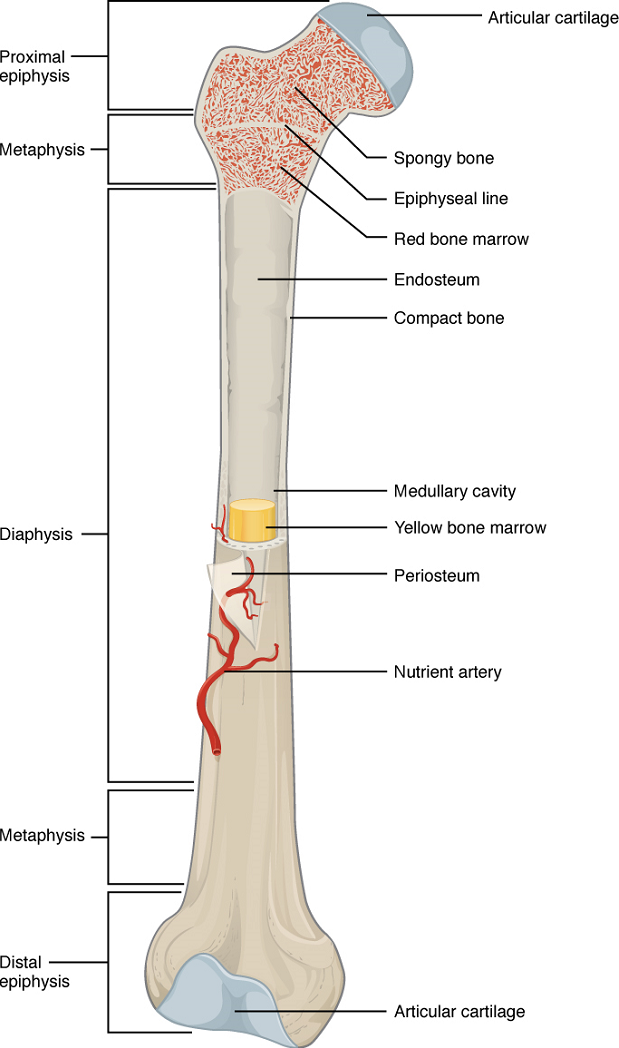
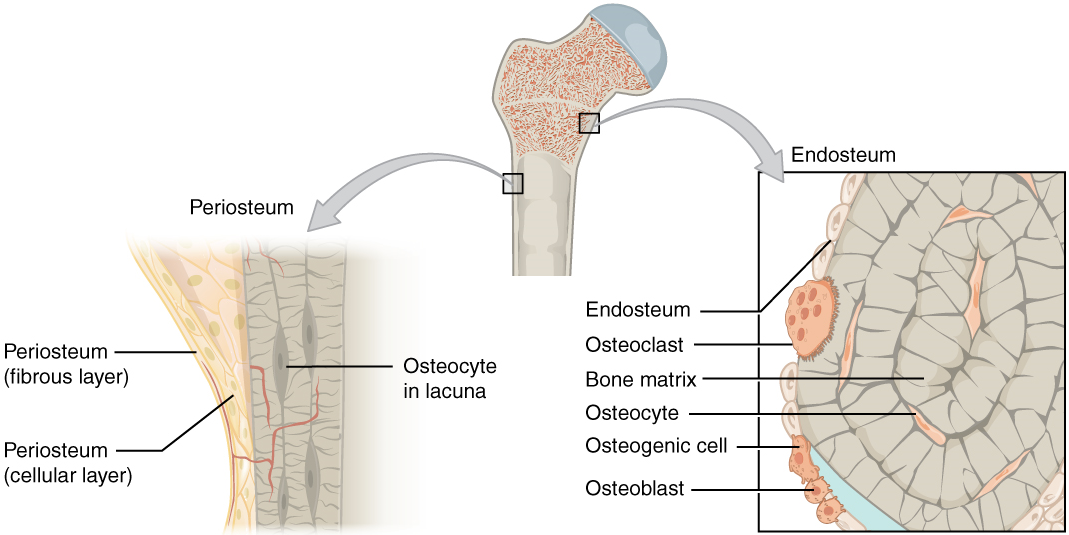
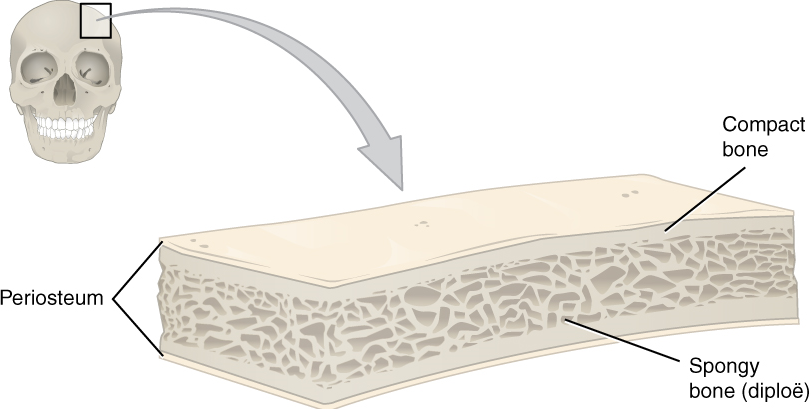
Flat bones, like those of the cranium, consist of a layer of diploë (spongy bone), lined on either side by a layer of compact bone (Figure 10.7). The two layers of compact bone and the interior spongy bone work together to protect the internal organs. If the outer layer of a cranial bone fractures, the brain is still protected by the intact inner layer.
Bone Cells and Tissue
Bone contains a relatively small number of cells entrenched in a matrix of collagen fibers that provide a surface for inorganic salt crystals to adhere. These salt crystals, made of a substance called hydroxyapatite, form when calcium phosphate and calcium carbonate combine with other inorganic salts and solidify (i.e., calcify) on the collagen fibers. The crystals give bones their hardness and strength, while the collagen fibers give them flexibility.
Although bone cells compose a small amount of the bone volume, they are crucial to the function of bones. Four types of cells are found within bone tissue: osteoblasts, osteocytes, osteogenic cells, and osteoclasts (Figure 10.8).
The osteoblast is the bone cell responsible for forming new bone and is found in the growing portions of bone, including the periosteum and endosteum. Osteoblasts, which do not divide, synthesize and secrete the collagen matrix and calcium salts. As the secreted matrix surrounding the osteoblast calcifies, the osteoblast becomes trapped within it; as a result, it changes in structure and becomes an osteocyte, the primary cell of mature bone and the most common type of bone cell. Each osteocyte is located in a space called a lacuna and is surrounded by bone matrix. Osteocytes maintain the mineral concentration of the matrix. Like osteoblasts, osteocytes lack mitotic activity. They can communicate with each other and receive nutrients via long cytoplasmic processes that extend through canaliculi (singular = canaliculus), channels within the bone matrix.
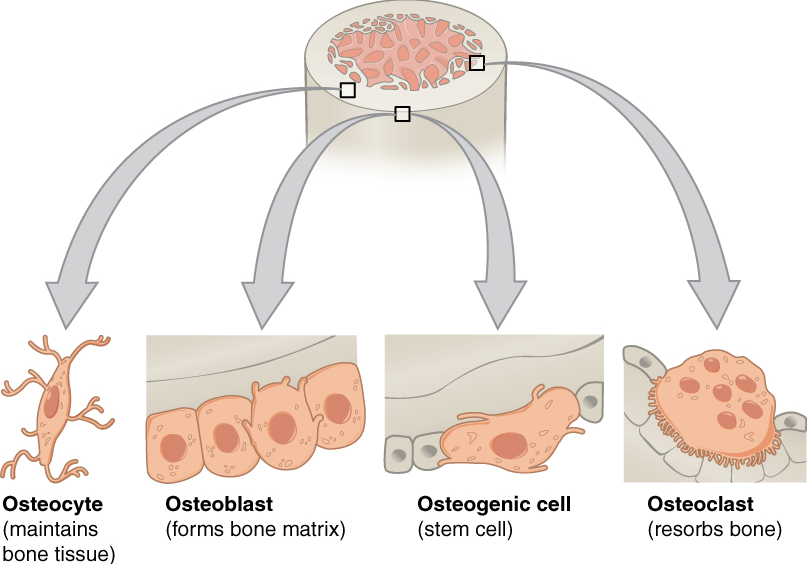
If osteoblasts and osteocytes are incapable of mitosis, then how are they replenished when old ones die? The answer lies in the properties of a third category of bone cells—the osteogenic cell. These cells are undifferentiated with high mitotic activity; and they are the only bone cells that divide. Immature osteogenic cells are found in the deep layers of the periosteum and the marrow. They differentiate and develop into osteoblasts.
The dynamic nature of bone means that new tissue is constantly formed, and old, injured, or unnecessary bone is dissolved for repair or for calcium release. The cell responsible for bone resorption, or breakdown, is the osteoclast. They are found on bone surfaces, are multinucleated, and originate from monocytes and macrophages, two types of white blood cells, rather than from osteogenic cells. Osteoclasts are continually breaking down old bone, while osteoblasts are continually forming new bone. The ongoing balance between osteoblasts and osteoclasts is responsible for the constant but subtle reshaping of bone. Table 10.2 reviews the bone cells, their functions, and locations.
Cell type | Function | Location |
---|---|---|
Osteogenic cells | Develop into osteoblasts | Deep layers of the periosteum and the marrow |
Osteoblasts | Bone formation | Growing portions of bone, including periosteum and endosteum |
Osteocytes | Maintain mineral concentration of matrix | Entrapped in matrix (in lacunae) |
Osteoclasts | Bone resorption | Bone surfaces and at sites of old, injured, or unneeded bone |
Compact and Spongy Bone
The differences between compact and spongy bone are best explored via their histology. Most bones contain compact and spongy osseous tissue, but their distribution and concentration vary based on the bone’s overall function. Compact bone is dense so that it can withstand compressive forces, while spongy (cancellous) bone has open spaces and supports shifts in weight distribution.
1. Compact Bone: Compact bone is the denser, stronger of the two types of bone tissue (Figure 10.9). It can be found deep to the periosteum and in the diaphyses of long bones, where it provides support and protection.
The microscopic structural unit of compact bone is called an osteon, or Haversian system. Each osteon is composed of concentric rings of calcified matrix called lamellae (singular = lamella). Running down the center of each osteon is the central canal, or Haversian canal, which contains blood vessels, nerves, and lymphatic vessels. These vessels and nerves branch off at right angles through a perforating canal, also known as Volkmann’s canals, to extend to the periosteum and endosteum.
The osteocytes are located inside spaces called lacunae (singular = lacuna), found at the borders of adjacent lamellae. As described earlier, canaliculi connect with the canaliculi of other lacunae and eventually with the central canal. This system allows nutrients to be transported to the osteocytes and wastes to be removed from them.
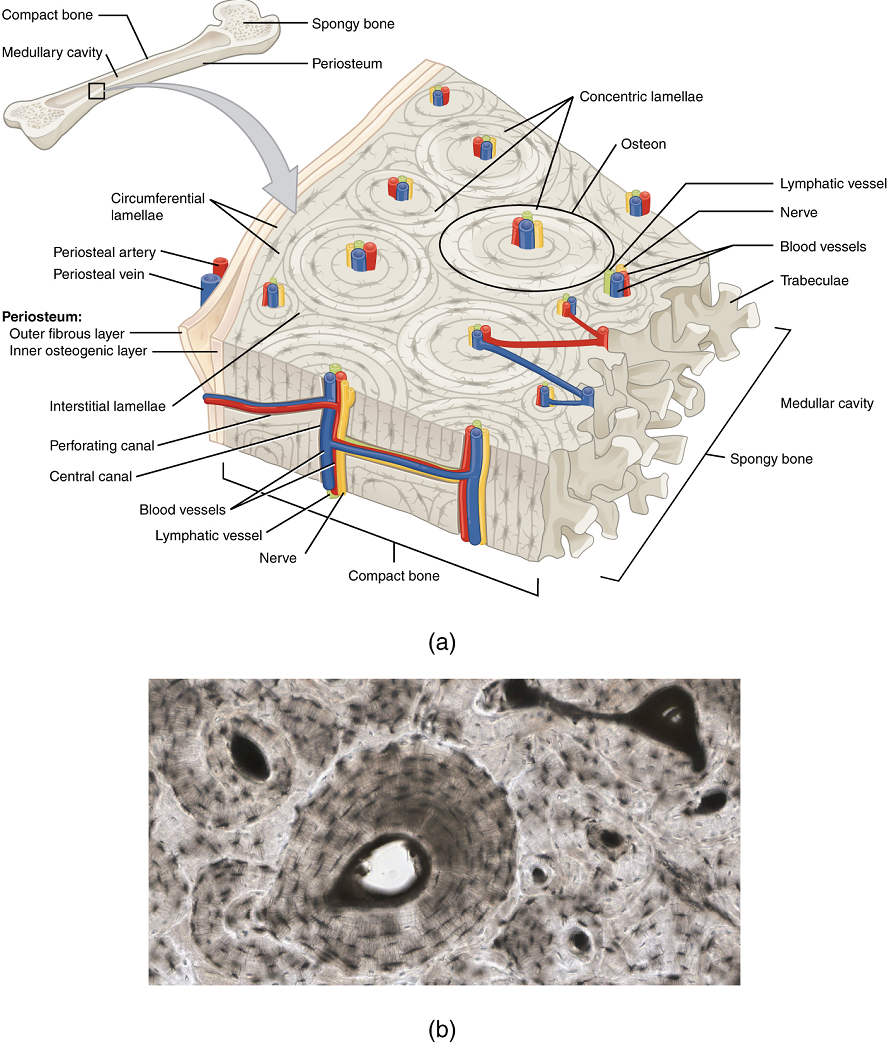
2. Spongy (Cancellous) Bone: Like compact bone, spongy bone, also known as cancellous bone, contains osteocytes housed in lacunae, but they are not arranged in concentric circles. Instead, the lacunae and osteocytes are found in a lattice-like network of matrix spikes called trabeculae (singular = trabecula; Figure 10.10). The trabeculae may appear to be a random network, but each trabecula forms along lines of stress to provide strength to the bone. The spaces of the trabeculated network provide balance to the dense and heavy compact bone by making bones lighter so that muscles can move them more easily. In addition, the spaces in some spongy bones contain red marrow, protected by the trabeculae, where hematopoiesis occurs.
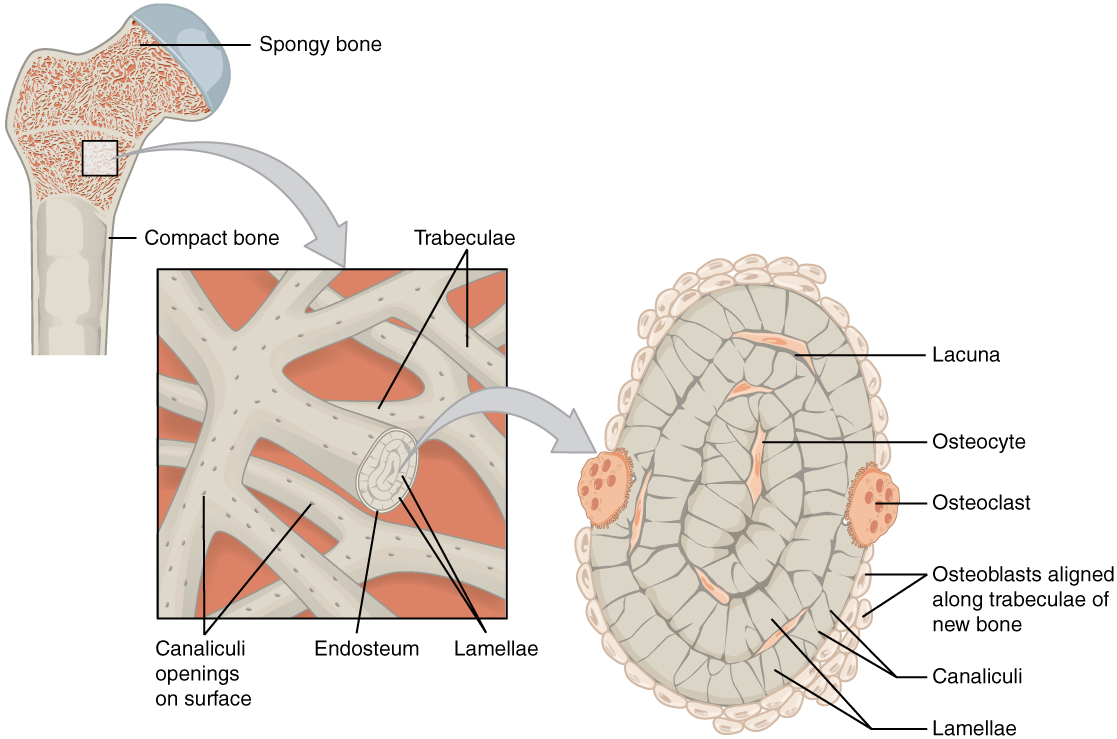
Blood and Nerve Supply
The spongy bone and medullary cavity receive nourishment from arteries that pass through the compact bone. The arteries enter through the nutrient foramen (plural = foramina), a small opening in the diaphysis (Figure 10.11). The osteocytes in spongy bone are nourished by blood vessels of the periosteum that penetrate spongy bone and blood that circulates in the marrow cavities. As the blood passes through the marrow cavities, it is collected by veins, which then pass out of the bone through the foramen.
In addition to the blood vessels, nerves follow the same paths into the bone where they tend to concentrate in the more metabolically active regions of the bone. The nerves sense pain, and it appears they also play roles in regulating blood supplies and in bone growth, hence their concentrations in metabolically active sites of the bone.
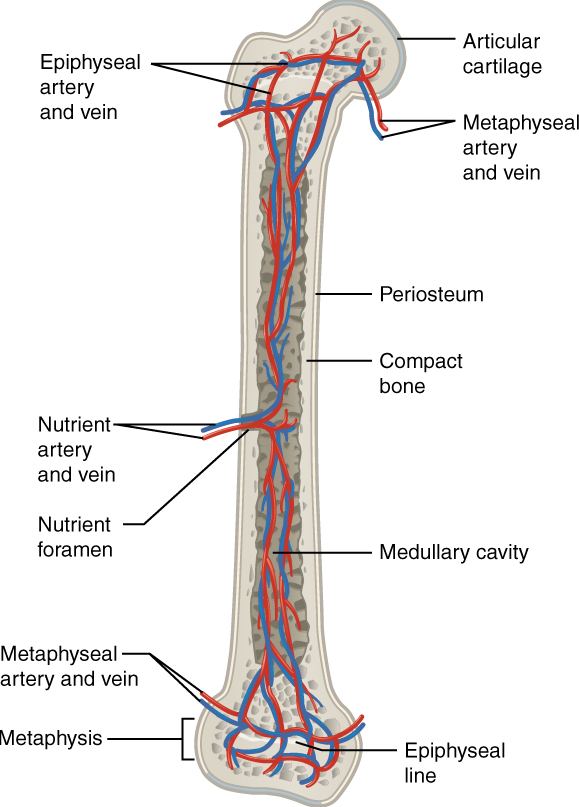
Test Your Knowledge
I. Describe the structure and histology of the skeletal system.
- Based on previously covered material and the information in this unit, describe each of the different cell types found in cartilage and bone. For each cell type, identify:
- Where in the body, and from which type of cell, it arose.
- Where it normally resides in the body, as specifically as possible.
- What its main function is, and how (briefly) it serves that function.
- What happens to the cell if the matrix that surrounds it calcifies.
- Compare and contrast the components of cartilage matrix and bone matrix, explaining the differences in the physical characteristics of cartilage and bone.
- From what tissue type do bones and cartilage arise during early development? What other mature tissues arise from the same fetal tissue type?
II. Define and identify the following parts of a long bone: diaphysis, epiphysis, metaphysis, articular cartilage, periosteum, medullary cavity, and endosteum.
- Identify a typical long bone, showing the main external and internal features and identifying all the main tissue types found in a long bone.
III. Compare the composition and function of compact bone versus spongy bone.
- Compare and contrast compact bone and spongy bone, in terms of the following characteristics:
- The tissue type and cell type found in each type of bone.
- The arrangement of tissue and/or cells in each type of bone.
- The location of each within a bone.
- The function of each type of bone.
Part 4: Bone Formation and Development
In the early stages of embryonic development, the embryo’s skeleton consists of fibrous membranes and hyaline cartilage. By the sixth or seventh week of embryonic life, the actual process of bone development, ossification (osteogenesis), begins. There are two osteogenic pathways—intramembranous ossification and endochondral ossification—but bone is the same regardless of the pathway that produces it.
Cartilage Templates
Bone is a replacement tissue; that is, it uses a model tissue on which to lay down its mineral matrix. For skeletal development, the most common template is cartilage. During fetal development, a framework is laid down that determines where bones will form. This framework is a flexible, semi-solid cartilage matrix produced by chondroblasts. As the matrix surrounds and isolates chondroblasts, they mature into cells called chondrocytes. Unlike most connective tissues, cartilage is avascular, meaning that it has no blood vessels supplying nutrients and removing metabolic wastes. All of these functions are carried on by diffusion through the matrix. This is why damaged cartilage does not repair itself as readily as most tissues do.
Throughout fetal development and into childhood growth and development, bone forms on the cartilaginous matrix. By the time a fetus is born, most of the cartilage has been replaced with bone. Some additional cartilage will be replaced throughout childhood, and some cartilage remains in the adult skeleton.
Intramembranous Ossification
During intramembranous ossification, compact and spongy bone develops directly from sheets of mesenchymal (undifferentiated) connective tissue. The flat bones of the face, most of the cranial bones, and the clavicles (collarbones) are initially formed via intramembranous ossification.
The process begins when mesenchymal cells in the embryonic skeleton gather together and begin to differentiate into specialized cells (Figure 10.12a). Some of these cells will form capillaries, while others will become osteogenic cells and then osteoblasts. Although they will ultimately be spread out by the formation of bone tissue, early osteoblasts appear in a cluster called an ossification center.
The osteoblasts secrete osteoid, uncalcified matrix, which calcifies (hardens) within a few days as mineral salts are deposited on it, thereby entrapping the osteoblasts within. Once entrapped, the osteoblasts become osteocytes (Figure 10.12b). As osteoblasts transform into osteocytes, osteogenic cells in the surrounding connective tissue differentiate into new osteoblasts.
Osteoid (unmineralized bone matrix) secreted around the capillaries results in a trabecular matrix, while osteoblasts on the surface of the spongy bone become the periosteum (Figure 10.12c). The periosteum then creates a protective layer of compact bone superficial to the trabecular bone. The trabecular bone crowds nearby blood vessels, which eventually condense into red marrow (Figure 10.12d).
Intramembranous ossification begins in utero during fetal development and continues on into adolescence. At birth, the skeleton is not fully ossified. Most joints of the skull, for example, are more mobile in an infant than in an adult to allow the skull to deform during passage through the birth canal. The flat bones of the cranium continue to grow throughout childhood, ultimately being separated by narrow immobile joints called sutures. Each clavicle also initially (at about six weeks of embryonic age) forms by intramembranous ossification from two primary ossification centers that fuse together in utero to form a single bone with cartilage at both ends. This cartilage later ossifies to form the mature clavicles with articular cartilage on either end (usually in an individual’s early twenties). The last bones to ossify via intramembranous ossification are the flat bones of the face, which reach their adult size at the end of the adolescent growth spurt. The mandible in an infant, for example, consists of two separate bones (left and right), connected by a joint called a symphysis. This mandibular symphysis is fully ossified within the first year of life, permanently fusing the left and right bones to form the mandible.
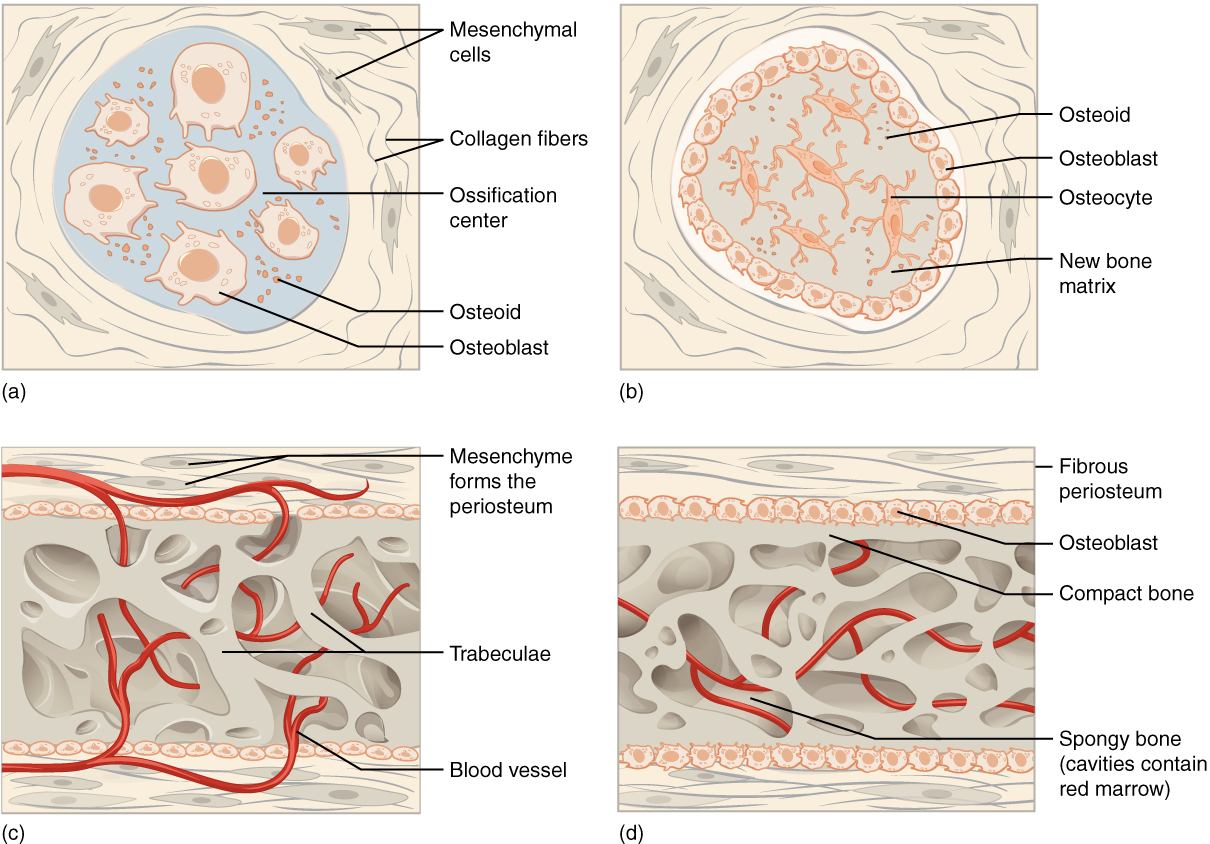
Endochondral Ossification
In endochondral ossification, bone develops by replacing hyaline cartilage. Cartilage does not become bone but instead serves as a template to be completely replaced by new bone. Endochondral ossification takes much longer than intramembranous ossification. Bones at the base of the skull and long bones form via endochondral ossification.
In a long bone, for example, at about six to eight weeks after conception, some of the mesenchymal cells differentiate into chondroblasts (cells that secrete the organic components of cartilage matrix) that form the cartilaginous skeletal precursor of the bones (Figure 10.13a). Soon after, the perichondrium, a membrane that covers the cartilage, appears (Figure 10.13b).
As more matrix is produced, the chondrocytes in the center of the cartilaginous model grow in size. As the matrix calcifies, nutrients can no longer reach the chondrocytes. This results in their death and the disintegration of the surrounding cartilage. Blood vessels invade the resulting spaces, not only enlarging the cavities but also carrying osteogenic cells with them, many of which will become osteoblasts (Figure 10.13c). These enlarging spaces eventually combine to become the medullary cavity (Figure 10.13d).
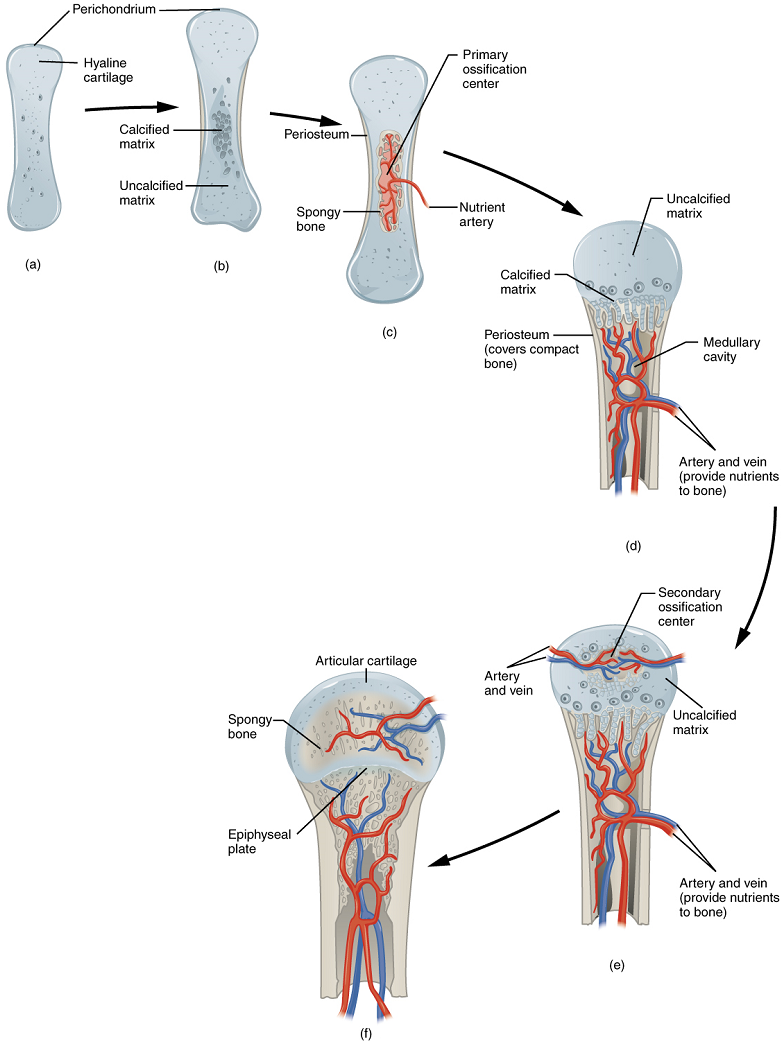
As the cartilage grows, capillaries penetrate it. This penetration initiates the transformation of the perichondrium into the bone-producing periosteum. Here, the osteoblasts form a periosteal collar of compact bone around the cartilage of the diaphysis. By the second or third month of fetal life, bone cell development and ossification ramps up and creates the primary ossification center, a region deep in the periosteal collar where ossification begins (Figure 10.13c).
While these deep changes are occurring, chondrocytes and cartilage continue to grow at the ends of the bone (the future epiphyses), which increases the bone’s length at the same time bone is replacing cartilage in the diaphyses. By the time the fetal skeleton is fully formed, cartilage only remains at the joint surface as articular cartilage and between the diaphysis and epiphysis as the epiphyseal plate, the latter of which is responsible for the longitudinal growth of bones (Figure 10.13f). After birth, this same sequence of events (matrix mineralization, death of chondrocytes, invasion of blood vessels from the periosteum, and seeding with osteogenic cells that become osteoblasts) occurs in the epiphyseal regions, and each of these centers of activity is referred to as a secondary ossification center (Figure 10.13e).
How Bones Grow in Length
The epiphyseal plate is the area of growth in a long bone. It is a layer of hyaline cartilage where ossification occurs in immature bones. On the epiphyseal side of the epiphyseal plate, cartilage is formed. On the diaphyseal side, cartilage is ossified, and the diaphysis grows in length. The epiphyseal plate is composed of four zones of cells and activity (Figure 10.14). The reserve zone is the region closest to the epiphyseal end of the plate and contains small chondrocytes within the matrix. These chondrocytes do not participate in bone growth but secure the epiphyseal plate to the osseous tissue of the epiphysis.
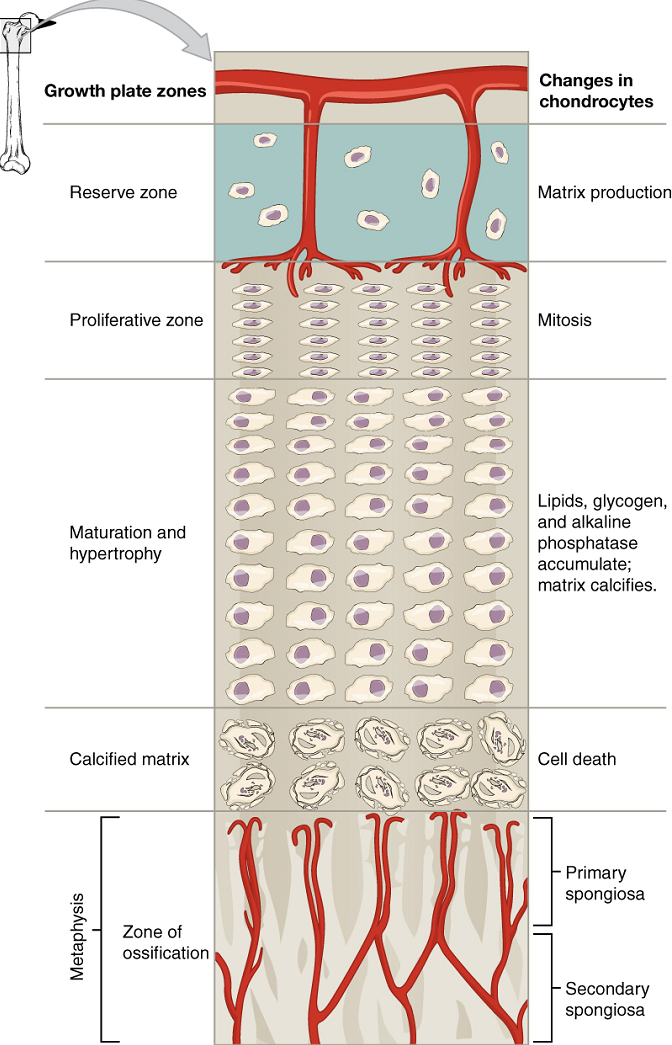
The proliferative zone is the next layer toward the diaphysis and contains stacks of slightly larger chondrocytes. It makes new chondrocytes (via mitosis) to replace those that die at the diaphyseal end of the plate. Chondrocytes in the next layer, the zone of maturation and hypertrophy, are older and larger than those in the proliferative zone. The more mature cells are situated closer to the diaphyseal end of the plate. The longitudinal growth of bone is a result of cellular division in the proliferative zone and the maturation of cells in the zone of maturation and hypertrophy.
Most of the chondrocytes in the zone of calcified matrix, the zone closest to the diaphysis, are dead because the matrix around them has calcified. Capillaries and osteoblasts from the diaphysis penetrate this zone, and the osteoblasts secrete bone tissue on the remaining calcified cartilage.
Thus, the zone of calcified matrix connects the epiphyseal plate to the diaphysis. A bone grows in length when osseous tissue is added to the diaphysis.
Bones continue to grow in length until early adulthood. The rate of growth is controlled by hormones, which will be discussed later. When the chondrocytes in the epiphyseal plate cease their proliferation and bone replaces the cartilage, longitudinal growth stops. All that remains of the epiphyseal plate is the now fully ossified epiphyseal line (Figure 10.15).
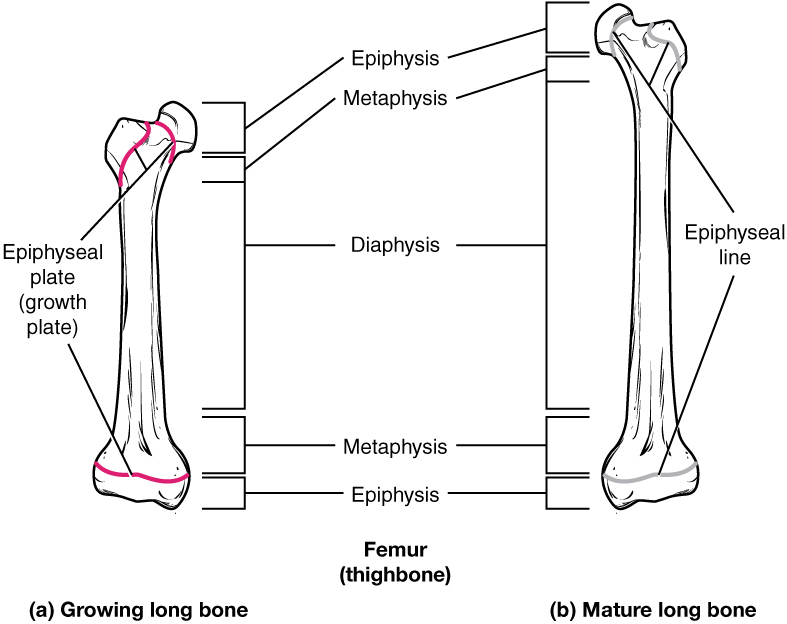
How Bones Grow in Diameter
While bones are increasing in length, they are also increasing in diameter; growth in diameter can continue even after longitudinal growth ceases. This is called appositional growth. Osteoclasts resorb old bone that lines the medullary cavity, while osteoblasts, via intramembranous ossification, produce new bone tissue beneath the periosteum. The erosion of old bone along the medullary cavity and the deposition of new bone beneath the periosteum not only increase the diameter of the diaphysis but also increase the diameter of the medullary cavity. This process is called modeling.
Bone Remodeling
The process in which matrix is resorbed on one surface of a bone and deposited on another is known as bone modeling. Modeling primarily takes place during a bone’s growth. However, in adult life, bone undergoes remodeling, in which resorption of old or damaged bone takes place on the same surface where osteoblasts lay new bone to replace that which is resorbed. Injury, exercise, and other activities lead to remodeling. Those influences are discussed later in the unit, but even without injury or exercise, about 5% to 10% of the skeleton is remodeled annually just by destroying old bone and renewing it with fresh bone.
Test Your Knowledge
I. Define ossification, compare intramembranous ossification with endochondral ossification, describe how a long bone grows in length and width, and specify how various factors might affect the rate of ossification and, by extension, the height of a mature individual.
II. Explain in detail the processes of:
- Intramembranous ossification
- Endochondral ossification
- Growth in length of a long bone
- Growth in width of a long bone
III. Compare and contrst the process of
- intramembranous ossification and endochondral ossification.
- endochondral ossification and lengthwise growth of a long bone.
- intramembranous ossification and widthwise growth of a long bone.
IV. The height of an individual is largely determined by the rate of ossification prior to physical maturity. Briefly explain why this is so.
Part 5: Fractures
A fracture is a broken bone. It will heal whether or not a physician resets it in its anatomical position. If the bone is not reset correctly, the healing process will keep the bone in its deformed position.
Types of Fractures: Fractures are classified by their complexity, location, and other features (Figure 10.16). Table 10.3 outlines common types of fractures. Some fractures may be described using more than one term because it may have the features of more than one type (e.g., an open transverse fracture). Of the types pictured in Figure 10.16 and Table 10.3, you are only required to understand the details of closed, open, comminuted, and greenstick fractures.
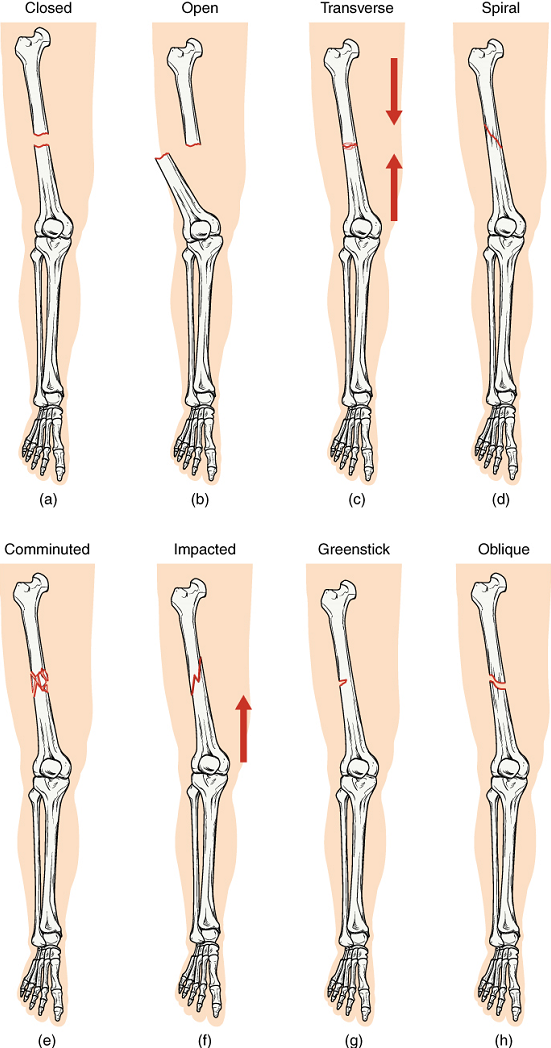
Type of fracture | Description |
---|---|
Transverse | Occurs straight across the long axis of the bone |
Oblique | Occurs at an angle that is not 90 degrees |
Spiral | Bone segments are pulled apart as a result of a twisting motion |
Comminuted | Several breaks result in many small pieces between two large segments |
Impacted | One fragment is driven into the other (usually a result of compression) |
Greenstick | A partial fracture in which only one side of the bone is broken |
Open (compound) | A fracture in which at least one end of the broken bone tears through the skin; carries a high risk of infection |
Closed (simple) | A fracture in which the skin remains intact |
Test Your Knowledge
I. Compare and contrast the common causes and appearance of:
- Closed (simple) vs. open (compound) fractures
- Comminuted vs. closed fractures
- Greenstick vs. closed fractures
- Comminuted vs. greenstick fractures
II. Can a fracture be classified as two of the above types at the same time? In other words, are any of the following fracture types possible? In each case, explain why or why not.
- A closed open fracture
- A closed comminuted fracture
- A closed greenstick fracture
- An open comminuted fracture
- An open greenstick fracture
B. Divisions of the Skeletal System
The skeletal system includes all of the bones, cartilages, and ligaments of the body that support and give shape to the body and body structures. The skeleton consists of the bones of the body. For adults, there are 206 bones in the skeleton. Younger individuals have higher numbers of bones because some bones fuse together during childhood and adolescence to form an adult bone. The skeleton is subdivided into two major divisions—the axial skeleton and the appendicular skeleton.
The Axial Skeleton: The skeleton is subdivided into two major divisions—the axial skeleton and appendicular skeleton. The axial skeleton forms the vertical central axis of the body and includes all bones of the head, neck, chest, and back (Figure 10.17). It serves to protect the brain, spinal cord, heart, and lungs. It also serves as the attachment site for muscles that move the head, neck, and back, and for muscles that act across the shoulder and hip joints to move their corresponding limbs.
The axial skeleton of the adult consists of 80 bones, including the skull, the vertebral column, and the thoracic cage. The skull is formed by 22 bones. Also associated with the head are an additional seven bones, including the hyoid bone and the ear ossicles (three small bones found in each middle ear). The vertebral column consists of 24 bones, each called a vertebra, plus the sacrum and coccyx. The thoracic cage includes the 12 pairs of ribs, and the sternum, the flattened bone of the anterior chest.
The Appendicular Skeleton: The appendicular skeleton includes all bones of the upper and lower limbs, plus the bones that attach each limb to the axial skeleton (Figure 10.17). There are 126 bones in the appendicular skeleton of an adult. The bones of the appendicular skeleton are covered later in the unit.
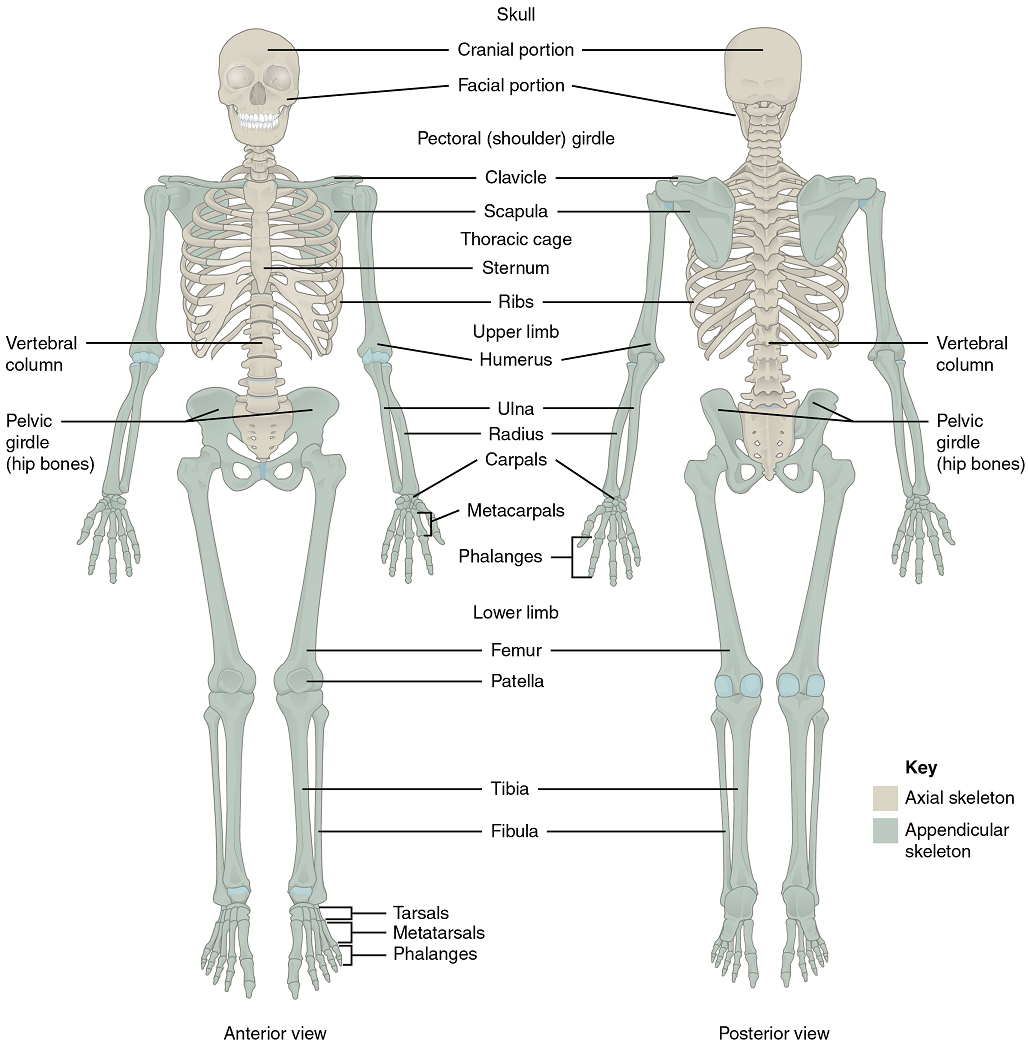
Part 1: The Axial Skeleton
The Skull
The cranium (skull) is the skeletal structure of the head that supports the face and protects the brain. It is subdivided into the facial bones and the braincase, or cranial vault (Figure 10.19). The facial bones underlie the facial structures, form the nasal cavity, enclose the eyeballs, and support the teeth of the upper and lower jaws. The rounded braincase surrounds and protects the brain and houses the middle and inner ear structures.
In the adult, the skull consists of 22 individual bones, 21 of which are immobile and united into a single unit. The 22nd bone is the mandible (lower jaw), which is the only moveable bone of the skull.
Development of the Skull: As the braincase bones grow in the fetal skull, they remain separated from each other by large areas of dense connective tissue, each of which is called a fontanelle (Figure 10.18). The fontanelles are the soft spots on an infant’s head. They are important during birth because these areas allow the skull to change shape as it squeezes through the birth canal. After birth, the fontanelles allow for continued growth and expansion of the skull as the brain enlarges. The largest fontanelle is located on the anterior head, at the junction of the frontal and parietal bones. The fontanelles decrease in size and disappear by age 2. However, the skull bones remained separated from each other at the sutures, which contain dense fibrous connective tissue that unites the adjacent bones. The connective tissue of the sutures allows for continued growth of the skull bones as the brain enlarges during childhood growth. This structure also means that, although the size of the cranium increases from birth to adulthood, proportionately, it does so less than other parts of the skeleton. The relative size of the cranium in proportion to the rest of the body therefore decreases with age from birth to adulthood.
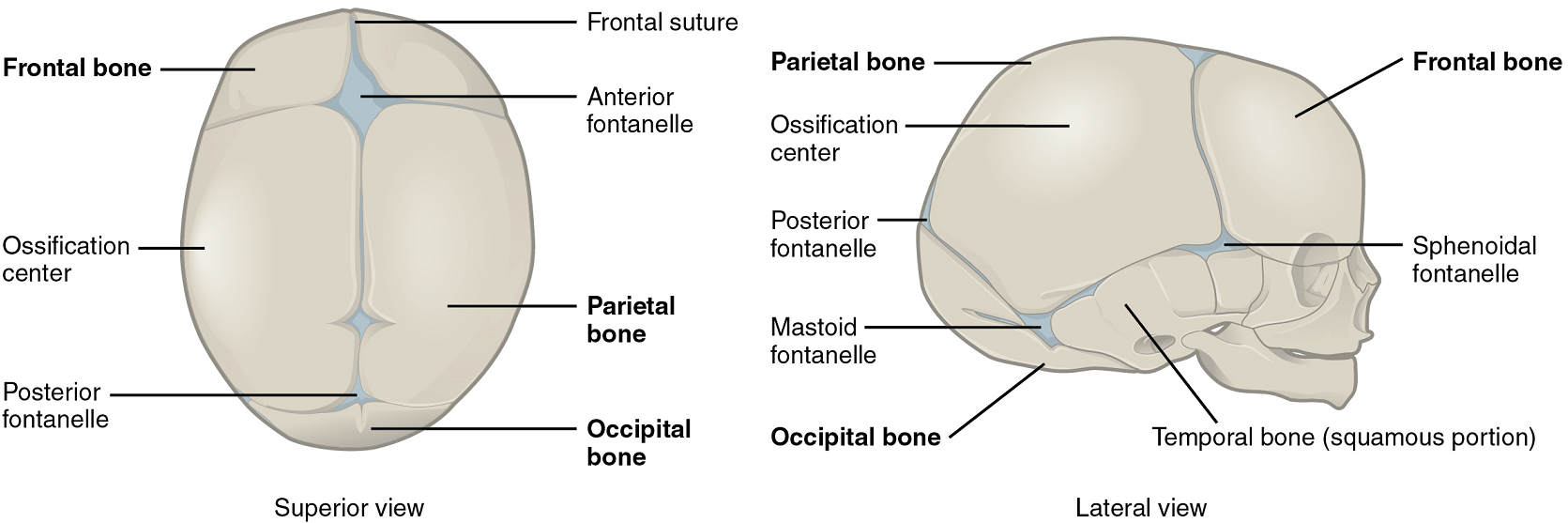
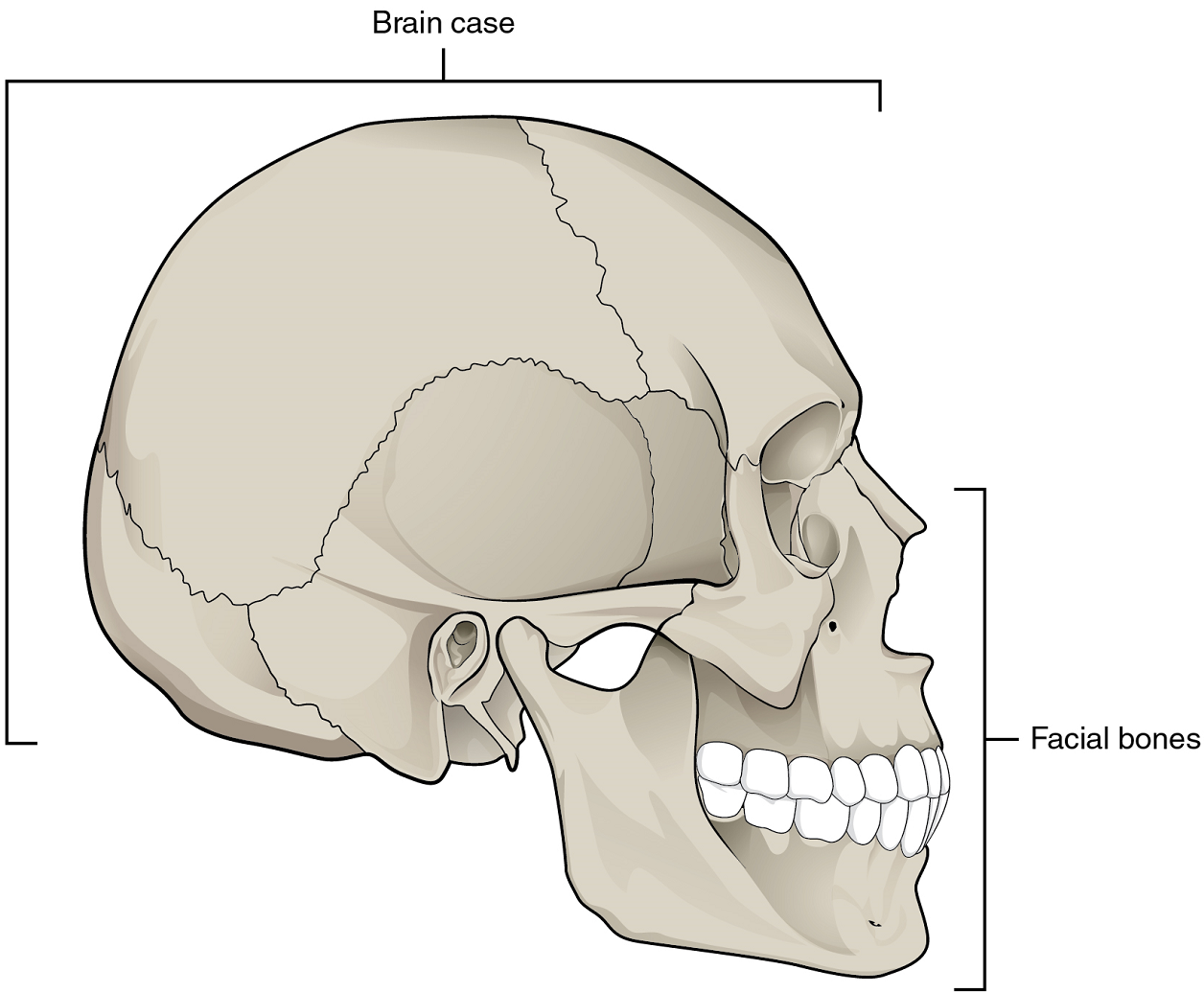
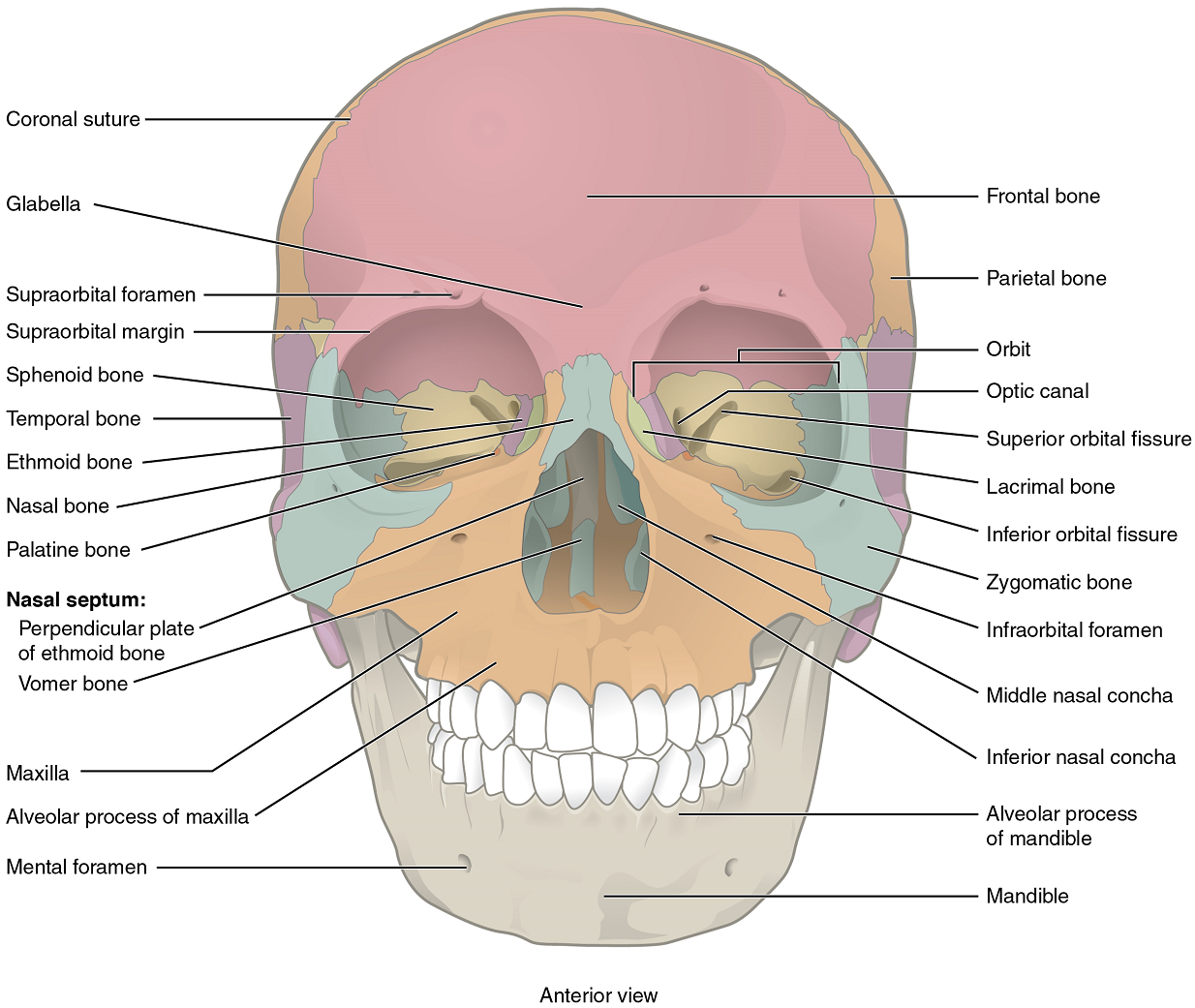
Bones of the Braincase: The braincase contains and protects the brain (Figure 10.19). The interior space that is almost completely occupied by the brain is called the cranial cavity.
The braincase consists of eight bones (Figures 10.20 & 10.21). These include the paired parietal and temporal bones, plus the unpaired frontal, occipital, sphenoid, and ethmoid bones. For our purposes, we will not be specifying the details of the sphenoid and ethmoid bones.
1. Parietal Bone: The parietal bone forms most of the upper lateral side of the skull (Figures 10.21 & 10.22). These are paired bones, with the right and left parietal bones joining together at the top of the skull. Each parietal bone is also bounded anteriorly by the frontal bone, inferiorly by the temporal bone, and posteriorly by the occipital bone.
2. Temporal Bone: The temporal bone forms the lower lateral side of the skull (Figure 10.21). Common wisdom has it that the temporal bone (temporal = “time”) is so named because this area of the head (the temple) is where hair typically first turns gray, indicating the passage of time.
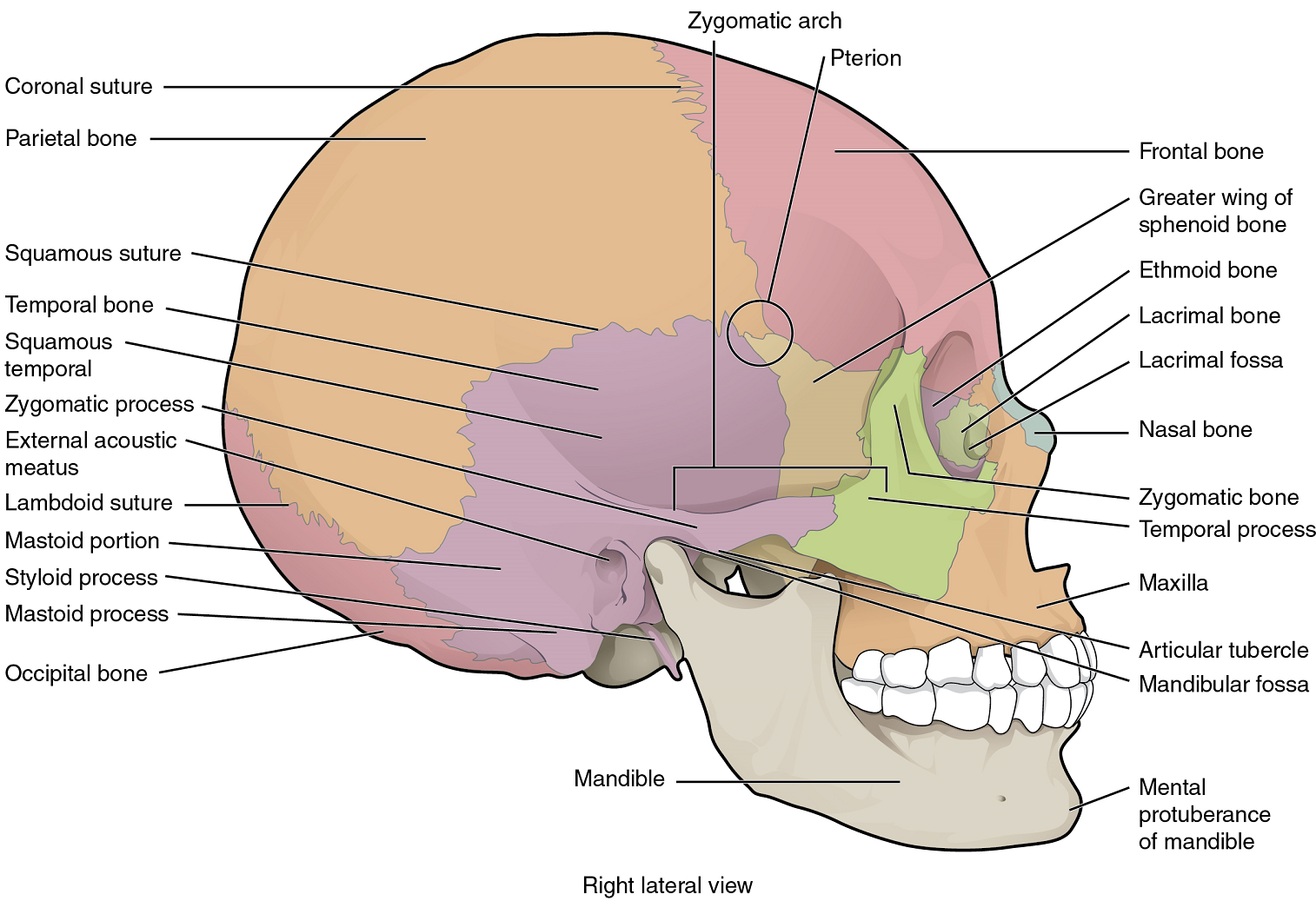
3. Frontal Bone: The frontal bone is the single bone that forms the forehead (Figure 10.20).
4. Occipital Bone: The occipital bone is the single bone that forms the posterior skull and posterior base of the cranial cavity (Figures 10.21 & 10.22). On its outside surface, at the posterior midline, is a small protrusion called the external occipital protuberance, which serves as an attachment site for a ligament of the posterior neck.
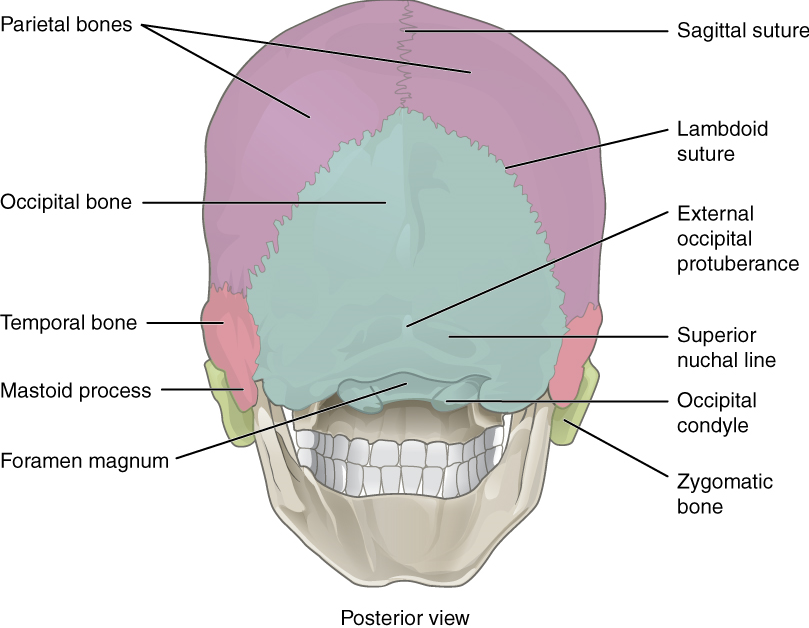
Facial Bones of the Skull: The facial bones of the skull form the upper and lower jaws, the nose, nasal cavity and nasal septum, and the orbit. The facial bones include 14 bones, with six paired bones and two unpaired bones (Figures 10.20 & 10.21). We will focus on the maxillary bones and the mandible bone.
1. Maxillary Bone: The maxillary bone, often referred to simply as the maxilla (plural = maxillae), is one of a pair that together form the upper jaw, much of the hard palate, the medial floor of the orbit, and the lateral base of the nose (Figures 10.20 & 10.21).
2. Mandible: The mandible forms the lower jaw and is the only moveable bone of the skull. At the time of birth, the mandible consists of paired right and left bones, but these fuse together during the first year to form the single U-shaped mandible of the adult skull (Figures 10.20 & 10.21).
The Bones of the Middle Ear: Three small bones (ossicles) are found on either side of the head in the middle ear. These are the malleus, incus, and stapes, and they function in transferring the vibrations from the eardrum (tympanic membrane) to the inner ear.
The Hyoid Bone: The hyoid bone is an independent bone that does not contact any other bone and thus is not part of the skull (Figure 10.23). It is a small U-shaped bone located in the upper neck near the level of the inferior mandible, with the tips of the “U” pointing posteriorly. The hyoid serves as the base for the tongue above and is attached to the larynx below and the pharynx posteriorly. The hyoid is held in position by a series of small muscles that attach to it either from above or below. These muscles act to move the hyoid up/down or forward/back. Movements of the hyoid are coordinated with movements of the tongue, larynx, and pharynx during swallowing and speaking.
The Vertebral Column
The vertebral column is also known as the spinal column or spine (Figure 10.24). It consists of a sequence of vertebrae (singular = vertebra), each of which is separated and united by an intervertebral disc. Together, the vertebrae and intervertebral discs form the vertebral column. It is a flexible column that supports the head, neck, and body and allows for their movements. It also protects the spinal cord, which passes down the back through openings in the vertebra.
Regions of the Vertebral Column: The vertebral column originally develops as a series of 33 vertebrae, but this number is eventually reduced to 24 vertebrae, plus the sacrum and coccyx. The vertebral column is subdivided into five regions, with the vertebrae in each area named for that region and numbered in descending order. In the neck, there are seven cervical vertebrae, each designated with the letter “C” followed by its number. Superiorly, the C1 vertebra articulates (forms a joint) with the occipital condyles of the skull. Inferiorly, C1 articulates with the C2 vertebra, and so on. Below these are the 12 thoracic vertebrae, designated T1–T12. The lower back contains the L1–L5 lumbar vertebrae. The single sacrum, which is also part of the pelvis, is formed by the fusion of five sacral vertebrae. Similarly, the coccyx, or tailbone, results from the fusion of four small coccygeal vertebrae. However, the sacral and coccygeal fusions do not start until age 20 and are not completed until middle age.
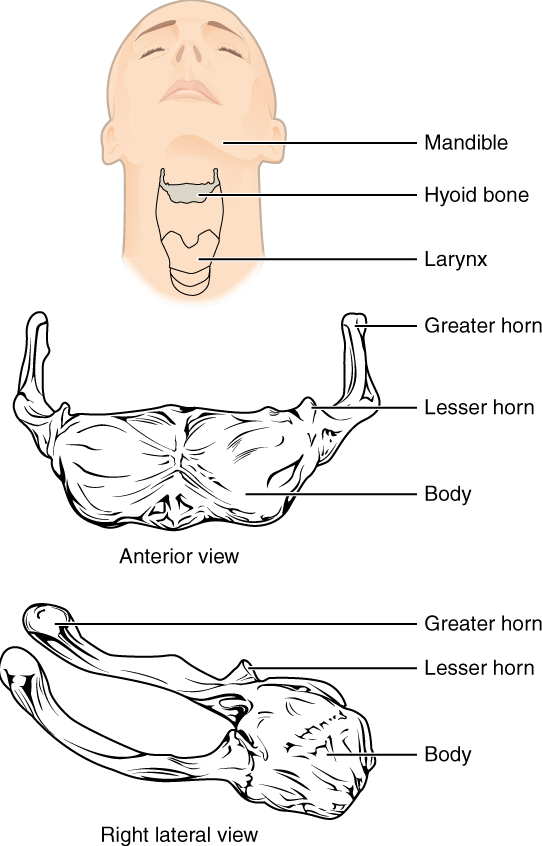
Curvatures of the Vertebral Column: The adult vertebral column does not form a straight line but instead has four curvatures along its length (see Figure 10.24). These curves increase the vertebral column’s strength, flexibility, and ability to absorb shock.
During fetal development, the body is flexed anteriorly into the fetal position, giving the entire vertebral column a single curvature that is concave anteriorly. In the adult, this fetal curvature is retained in two regions of the vertebral column as the thoracic curve, which involves the thoracic vertebrae, and the sacrococcygeal curve, formed by the sacrum and coccyx.
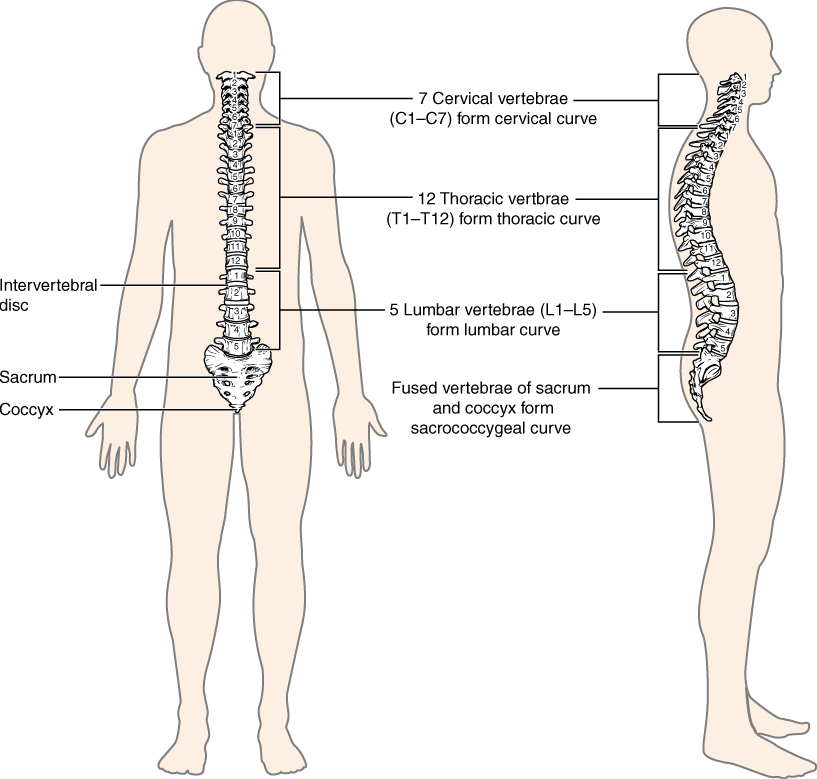
General Structure of a Vertebra: Within the different regions of the vertebral column, vertebrae vary in size and shape, but they all follow a similar structural pattern. A typical vertebra will consist of a body, a vertebral arch, and seven processes (Figure 10.25).
The body is the anterior portion of each vertebra and is the part that supports the body weight. Because of this, the vertebral bodies progressively increase in size and thickness going down the vertebral column. The bodies of adjacent vertebrae are separated and strongly united by an intervertebral disc.
The vertebral arch forms the posterior portion of each vertebra.
The large opening between the vertebral arch and body is the vertebral foramen, which contains the spinal cord. In the intact vertebral column, the vertebral foramina of all of the vertebrae align to form the vertebral (spinal) canal, which serves as the bony protection and passageway for the spinal cord down the back. When the vertebrae are aligned together in the vertebral column, notches in the margins of the pedicles of adjacent vertebrae together form an intervertebral foramen, the opening through which a spinal nerve exits from the vertebral column (Figure 10.26).
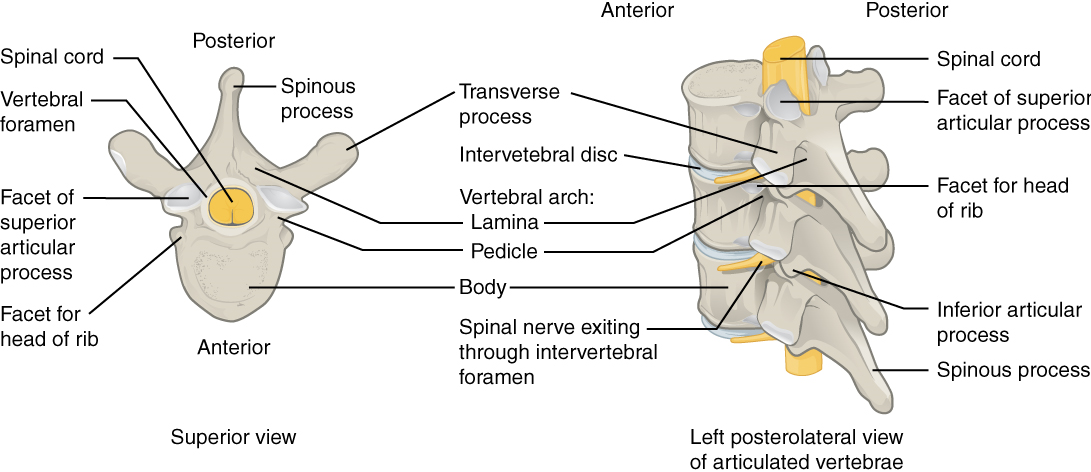
Seven processes arise from the vertebral arch. Each paired transverse process projects laterally and arises from the junction point between the pedicle and lamina. The single spinous process (vertebral spine) projects posteriorly at the midline of the back. The vertebral spines can easily be felt as a series of bumps just under the skin down the middle of the back. The transverse and spinous processes serve as important muscle attachment sites. A superior articular process extends or faces upward, and an inferior articular process faces or projects downward on each side of a vertebrae. The paired superior articular processes of one vertebra join with the corresponding paired inferior articular processes from the next higher vertebra. These junctions form slightly moveable joints between the adjacent vertebrae. The shape and orientation of the articular processes vary in different regions of the vertebral column and play a major role in determining the type and range of motion available in each region.
Regional Modifications of Vertebrae: In addition to the general characteristics of a typical vertebra described above, vertebrae also display characteristic size and structural features that vary between the different vertebral column regions. Thus, cervical vertebrae are smaller than lumbar vertebrae due to differences in the proportion of body weight that each will support. Thoracic vertebrae have sites for rib attachment, and the vertebrae that give rise to the sacrum and coccyx have fused together into single bones. We will focus on the anatomically distinct natures of the first two cervical vertebrae, the atlas and the axis.
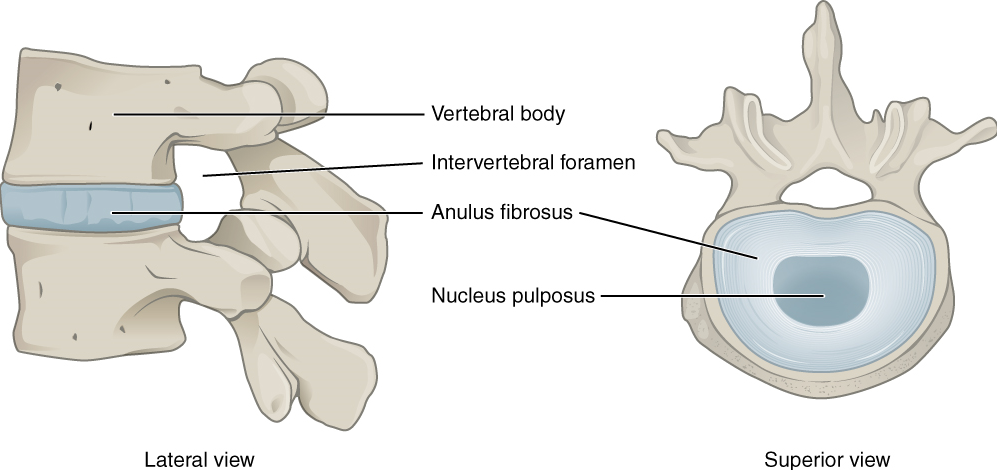
Cervical Vertebrae: Typical cervical vertebrae, such as C4 or C5, have several characteristic features that differentiate them from thoracic or lumbar vertebrae (Figure 10.27). Cervical vertebrae have a small body, reflecting the fact that they carry the least amount of body weight. Cervical vertebrae usually have a bifid (Y-shaped) spinous process. The transverse processes of the cervical vertebrae are sharply curved (U-shaped) to allow for passage of the cervical spinal nerves. Each transverse process also has an opening called the transverse foramen.
The first and second cervical vertebrae are further modified, giving each a distinctive appearance. The first cervical (C1) vertebra is also called the atlas, because this is the vertebra that supports the skull on top of the vertebral column (in Greek mythology, Atlas was the god who supported the heavens on his shoulders). The C1 vertebra does not have a body or spinous process. Instead, it is ring-shaped, consisting of an anterior arch and a posterior arch. The transverse processes of the atlas are longer and extend more laterally than do the transverse processes of any other cervical vertebrae. The superior articular processes face upward and are deeply curved for articulation with the occipital condyles on the base of the skull. The inferior articular processes are flat and face downward to join with the superior articular processes of the C2 vertebra.
The second cervical (C2) vertebra is called the axis, because it serves as the axis for rotation when turning the head right or left. The axis resembles typical cervical vertebrae in most respects but is easily distinguished by the dens (odontoid process), a bony projection that extends upward from the vertebral body. The dens joins with the inner aspect of the anterior arch of the atlas, where it is held in place by transverse ligament.
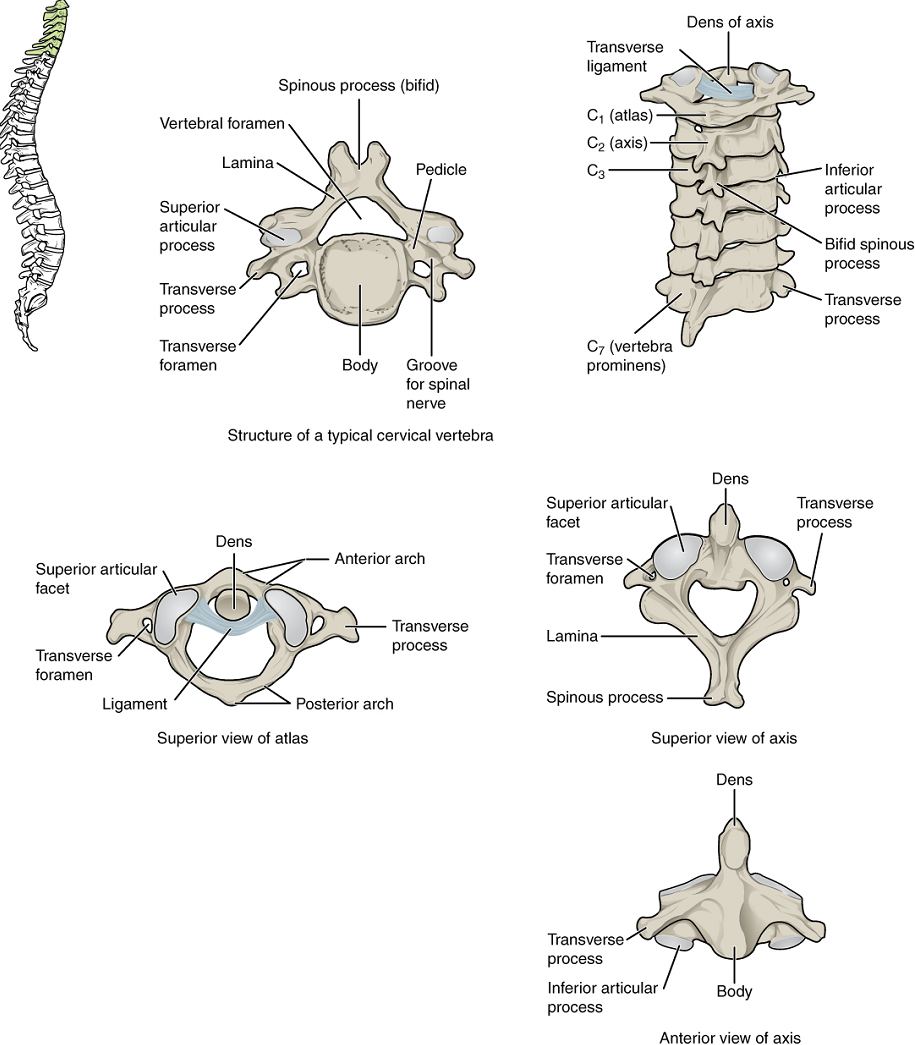
The Thoracic Cage
The thoracic cage (rib cage) forms the thorax (chest) portion of the body. It consists of the 12 pairs of ribs with their costal cartilages and the sternum (Figure 10.28). The ribs are anchored posteriorly to the 12 thoracic vertebrae (T1–T12). The thoracic cage protects the heart and lungs.
Sternum: The sternum is the elongated bony structure that anchors the anterior thoracic cage. It consists of three parts: the manubrium, body, and xiphoid process.
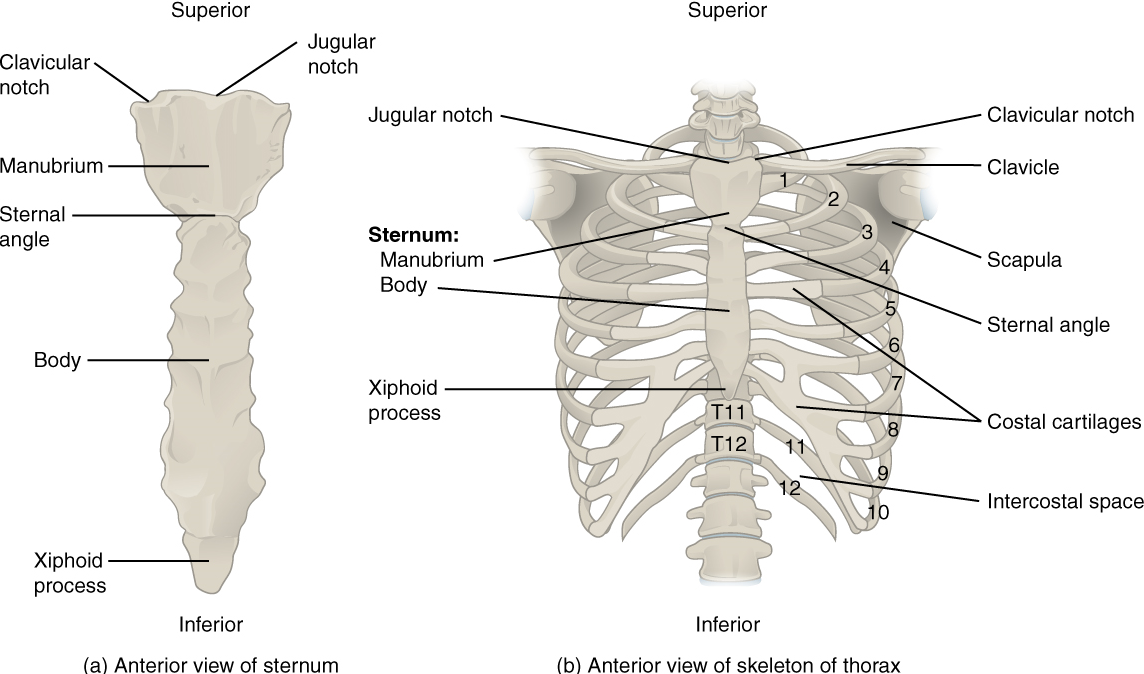
Ribs: Each rib is a curved, flattened bone that contributes to the wall of the thorax. The ribs articulate posteriorly with the T1–T12 thoracic vertebrae, and most attach anteriorly via their costal cartilages to the sternum. There are 12 pairs of ribs. The ribs are numbered 1–12 in accordance with the thoracic vertebrae.
The bony ribs do not extend anteriorly completely around to the sternum. Instead, each rib ends in a costal cartilage. These cartilages are made of hyaline cartilage and can extend for several inches. Most ribs are then attached, either directly or indirectly, to the sternum via their costal cartilage (Figure 10.28). The ribs are classified into three groups based on their relationship to the sternum.
Ribs 1–7 are classified as true ribs (vertebrosternal ribs). The costal cartilage from each of these ribs attaches directly to the sternum. Ribs 8–12 are called false ribs (vertebrochondral ribs). The costal cartilages from these ribs do not attach directly to the sternum. For ribs 8–10, the costal cartilages are attached to the cartilage of the next higher rib. Thus, the cartilage of rib 10 attaches to the cartilage of rib 9, rib 9 then attaches to rib 8, and rib 8 is attached to rib 7. The last two false ribs (11–12) are also called floating ribs (vertebral ribs). These are short ribs that do not attach to the sternum at all. Instead, their small costal cartilages terminate within the musculature of the lateral abdominal wall.
Test Your Knowledge
I. List all the components of the axial skeleton.
II. Specify the main functions of the axial skeleton. Explain how the overall shape of the axial skeleton is appropriate to its primary function.
III. Describe the structure and function of a typical vertebra and explain how these differ in the case of the atlas and axis.
Part 2. The Appendicular Skeleton
Attached to the axial skeleton are the limbs, whose 126 bones constitute the appendicular skeleton (Figure 10.29) These bones are divided into two groups: the bones that are located within the limbs themselves and the girdle bones that attach the limbs to the axial skeleton. The bones of the shoulder region form the pectoral girdle, which anchors the upper limb to the thoracic cage of the axial skeleton. The lower limb is attached to the vertebral column by the pelvic girdle.
Because of our upright stance, different functional demands are placed upon the upper and lower limbs. Thus, the bones of the lower limbs are adapted for weight-bearing support and stability, as well as for body locomotion via walking or running. In contrast, our upper limbs are not required for these functions. Instead, our upper limbs are highly mobile and can be utilized for a wide variety of activities. The large range of upper limb movements, coupled with the ability to easily manipulate objects with our hands and opposable thumbs, has allowed humans to construct the modern world in which we live.
The Pectoral Girdle
The bones that attach each upper limb to the axial skeleton form the pectoral girdle (shoulder girdle). This consists of two bones, the scapula and clavicle (Figure 10.30).
The clavicle (collarbone) is an S-shaped bone located on the anterior side of the shoulder. It is attached on its medial end to the sternum of the thoracic cage, which is part of the axial skeleton. The lateral end of the clavicle articulates (joins) with the scapula just above the shoulder joint. You can easily palpate, or feel with your fingers, the entire length of your clavicle.
The scapula (shoulder blade) lies on the posterior aspect of the shoulder. It is supported by the clavicle, which also articulates with the humerus (upper arm bone) to form the shoulder joint. The scapula is a flat, triangular-shaped bone with a prominent ridge running across its posterior surface. This ridge extends out laterally, where it forms the bony tip of the shoulder and joins with the lateral end of the clavicle. By following along the clavicle, you can palpate out to the bony tip of the shoulder, and from there, you can move back across your posterior shoulder to follow the ridge of the scapula. Move your shoulder around and feel how the clavicle and scapula move together as a unit. Both of these bones serve as important attachment sites for muscles that aid with movements of the shoulder and arm (Figures 10.30 & 10.31).
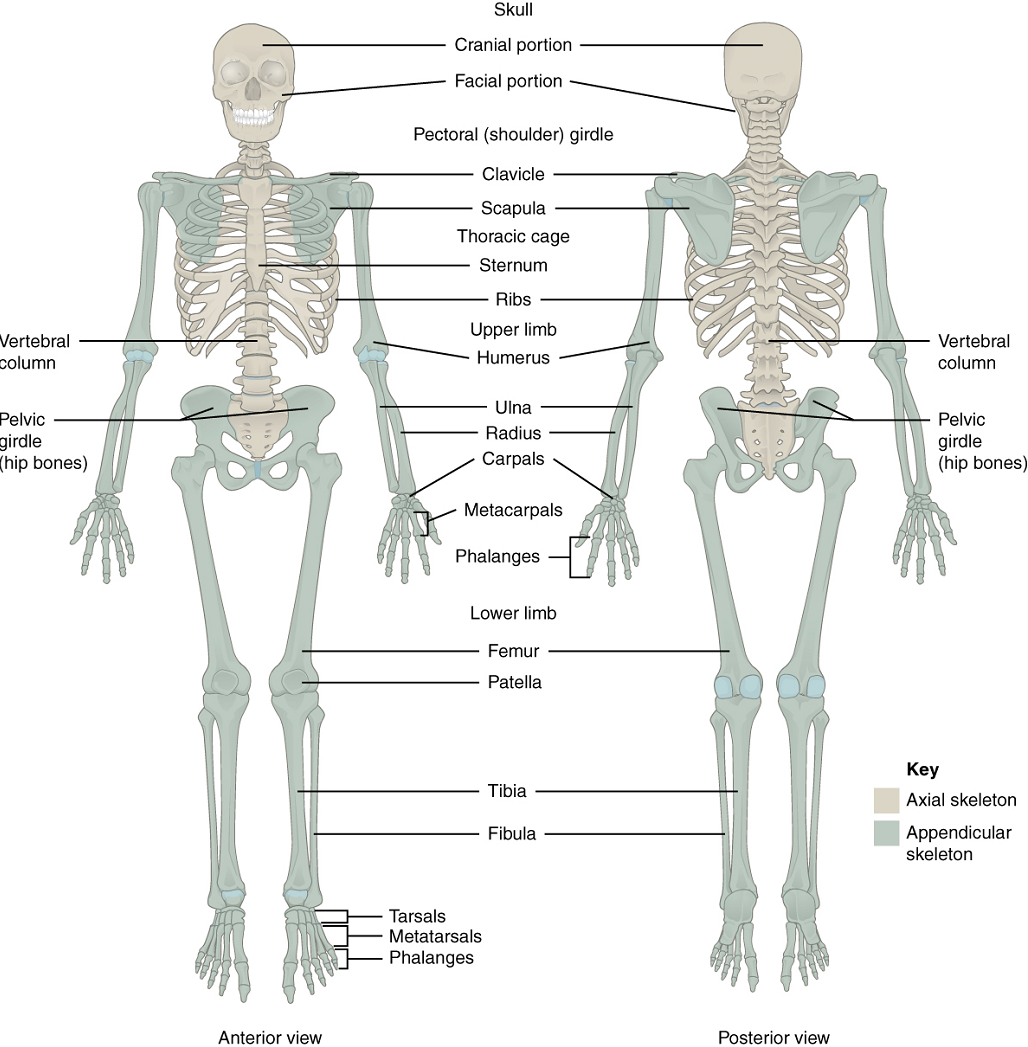
The right and left pectoral girdles are not joined to each other, allowing each to operate independently. In addition, the clavicle of each pectoral girdle is anchored to the axial skeleton by a single, highly mobile joint. This allows for the extensive mobility of the entire pectoral girdle, which in turn enhances movements of the shoulder and upper limb.
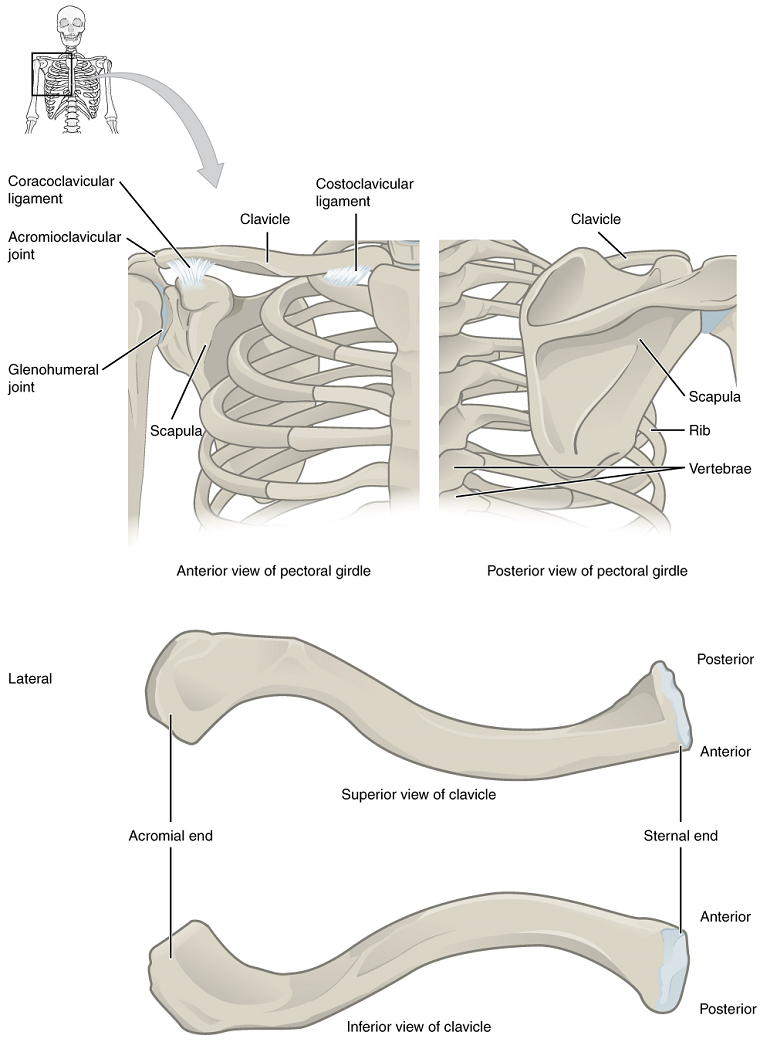
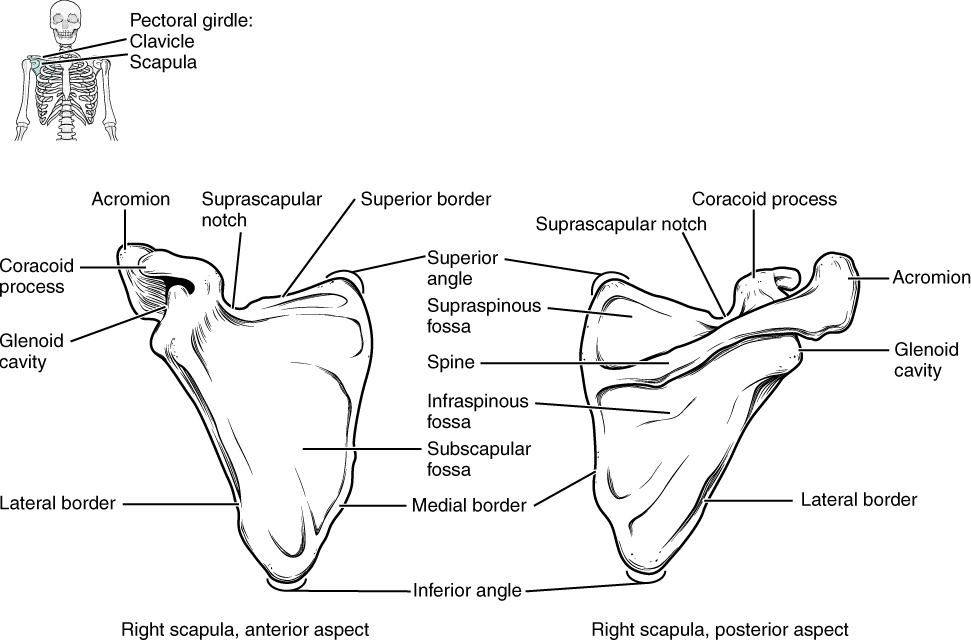
Bones of the Upper Limb
The upper limb is divided into three regions. These consist of the arm, located between the shoulder and elbow joints; the forearm, which is between the elbow and wrist joints; and the hand, which is located distal to the wrist. There are 30 bones in each upper limb. The humerus is the single bone of the upper arm, and the ulna (medially) and the radius (laterally) are the paired bones of the forearm. The base of the hand contains eight bones, each called a carpal bone, and the palm of the hand is formed by five bones, each called a metacarpal bone. The fingers and thumb contain a total of 14 bones, each of which is a phalanx bone of the hand (Figure 10.29).
Humerus: The humerus is the single bone of the upper arm region (Figure 10.32). At its proximal end is the head of the humerus. This is the large, round, smooth region that faces medially. The head articulates with the glenoid cavity of the scapula to form the glenohumeral (shoulder) joint. Distally, the humerus becomes flattened and has two articulation areas, which join the ulna and radius bones of the forearm to form the elbow joint.
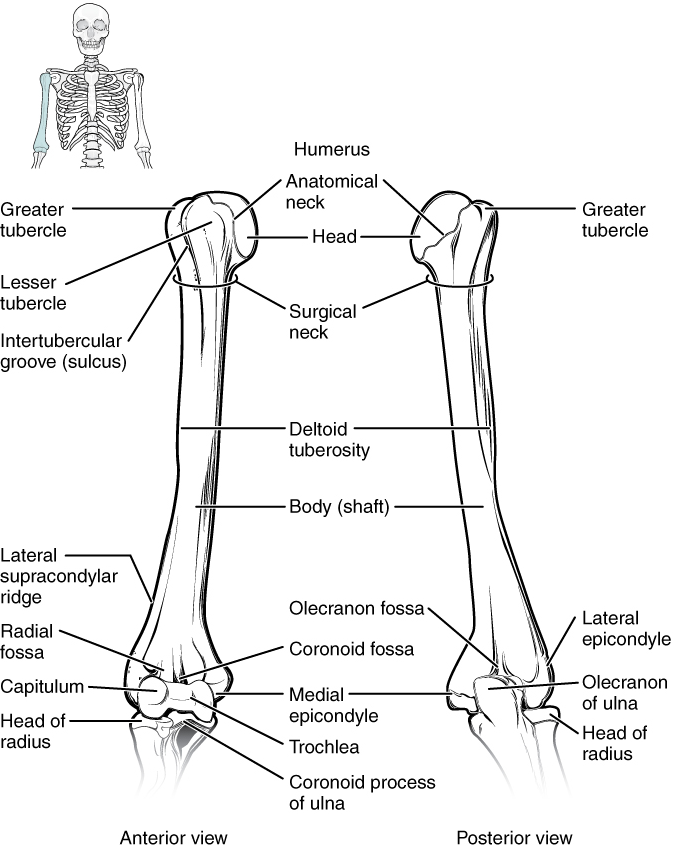
Ulna: The ulna is the medial bone of the forearm. It runs parallel to the radius, which is the lateral bone of the forearm (Figure 10.33). The proximal end of the ulna articulates with the humerus as part of the elbow joint.
Radius: The radius runs parallel to the ulna, on the lateral (thumb) side of the forearm (Figure 10.33). The head of the radius is a disc-shaped structure that forms the proximal end. The distal end of the radius has a smooth surface for articulation with two carpal bones to form the radiocarpal joint or wrist joint (Figures 10.34 & 10.35).
Carpal Bones: The wrist and base of the hand are formed by a series of eight small carpal bones (Figure 10.34). The carpal bones are arranged in two rows, forming a proximal row of four carpal bones and a distal row of four carpal bones.
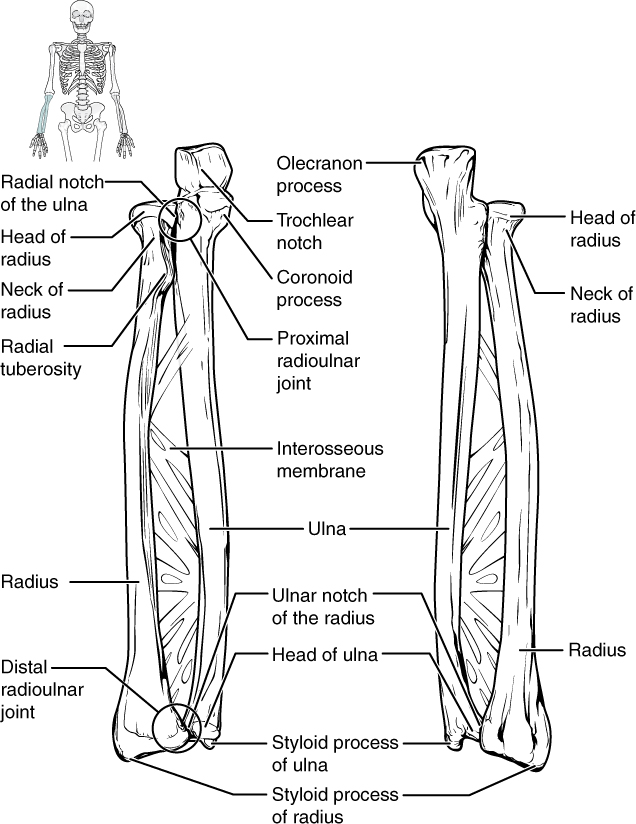
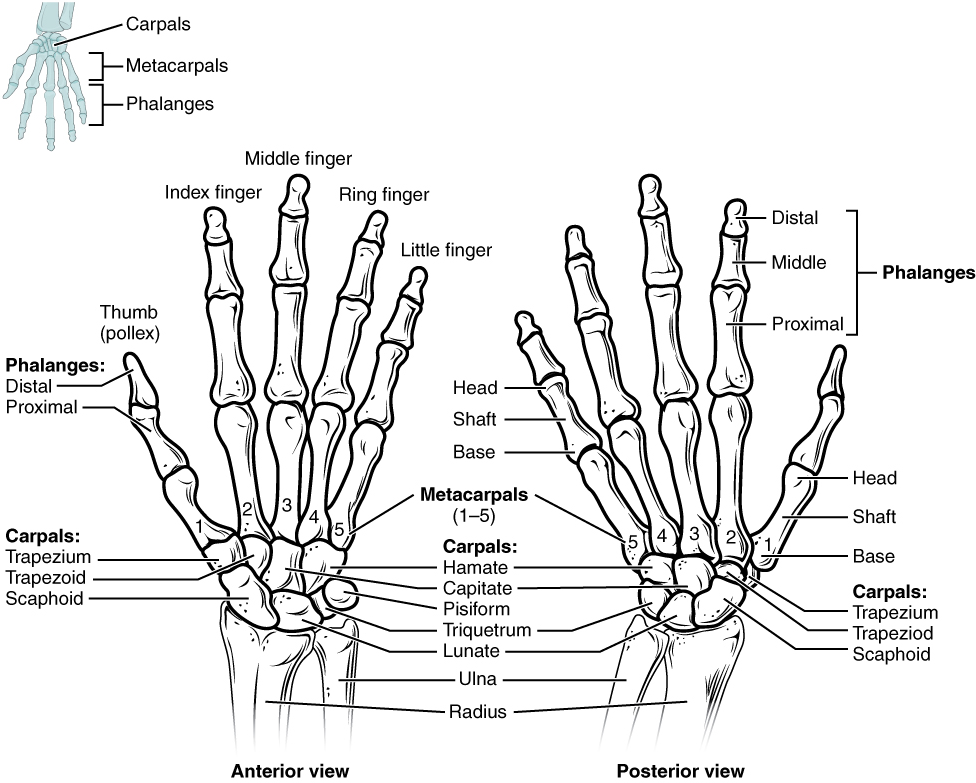
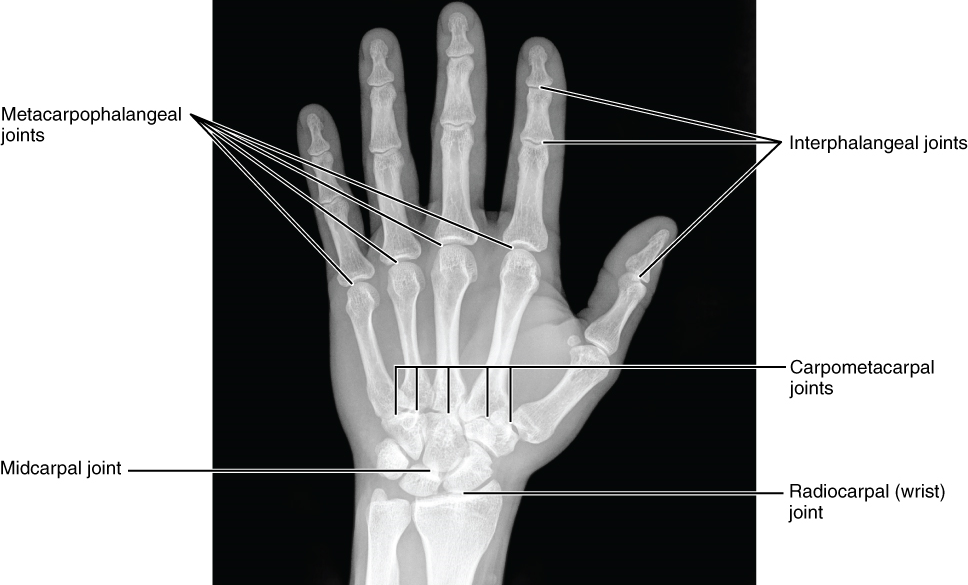
The carpal bones form the base of the hand. This can be seen in the radiograph (X-ray image) of the hand that shows the relationships of the hand bones to the skin creases of the hand (Figure 10.35).
Metacarpal Bones: The palm of the hand contains five elongated metacarpal bones. These bones lie between the carpal bones of the wrist and the bones of the fingers and thumb (Figure 10.34). The proximal end of each metacarpal bone articulates with one of the distal carpal bones. Each of these articulations is a carpometacarpal joint (Figure 10.35). The expanded distal end of each metacarpal bone articulates at the metacarpophalangeal joint with the proximal phalanx bone of the thumb or one of the fingers. The distal end also forms the knuckles of the hand, at the base of the fingers. The metacarpal bones are numbered 1–5, beginning at the thumb.
Phalanx Bones: The fingers and thumb contain 14 bones, each of which is called a phalanx bone (plural = phalanges), named after the ancient Greek phalanx (a rectangular block of soldiers). The thumb (pollex) is digit number 1 and has two phalanges, a proximal phalanx, and a distal phalanx bone (Figure 10.34). Digits 2 (index finger) through 5 (little finger) have three phalanges each, called the proximal, middle, and distal phalanx bones. An interphalangeal joint is one of the articulations between adjacent phalanges of the digits (Figure 10.35).
Test Your Knowledge
I. List all the components of the appendicular skeleton.
II. Specify the main functions of the appendicular skeleton. Explain how the overall shape of the appendicular skeleton is appropriate to its primary function.
Part 3: The Pelvic Girdle and Pelvis
The pelvic girdle (hip girdle) is formed by a single bone, the hip bone or coxal bone (coxal = “hip”), which serves as the attachment point for each lower limb. Each hip bone, in turn, is firmly joined to the axial skeleton via its attachment to the sacrum of the vertebral column. The right and left hip bones also converge anteriorly to attach to each other. The bony pelvis is the entire structure formed by the two hip bones, the sacrum and the coccyx, which is attached inferiorly to the sacrum (Figure 10.36).
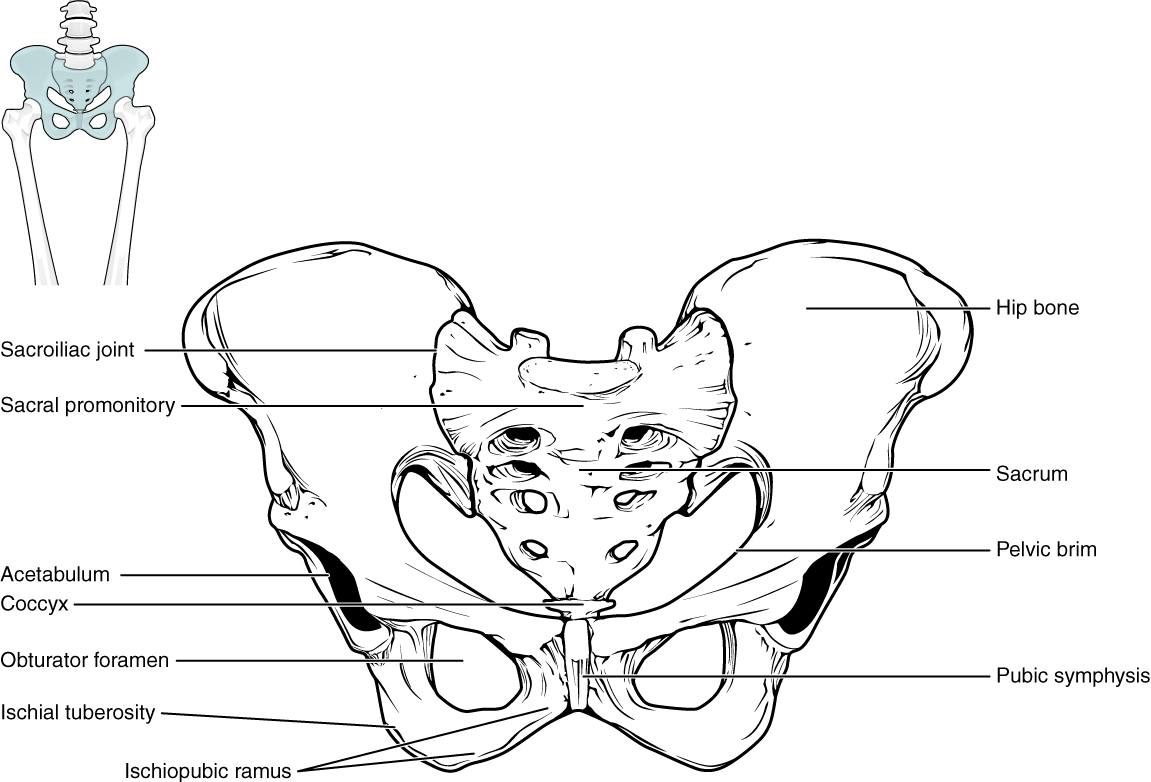
Unlike the bones of the pectoral girdle, which are highly mobile to enhance the range of upper limb movements, the bones of the pelvis are strongly united to each other to form a largely immobile, weight-bearing structure. This is important for stability because it enables the weight of the body to be easily transferred laterally from the vertebral column, through the pelvic girdle and hip joints, and into either lower limb whenever the other limb is not bearing weight. Thus, the immobility of the pelvis provides a strong foundation for the upper body as it rests on top of the mobile lower limbs.
Hip Bone: The hip bone, or coxal bone, forms the pelvic girdle portion of the pelvis. The paired hip bones are the large, curved bones that form the lateral and anterior aspects of the pelvis. Each adult hip bone is formed by three separate bones that fuse together during the late teenage years. These bony components are the ilium, ischium, and pubis (Figure 10.37). These names are retained and used to define the three regions of the adult hip bone.
The ilium is the fan-like, superior region that forms the largest part of the hip bone. It is firmly united to the sacrum at the largely immobile sacroiliac joint (Figure 10.36). The ischium forms the posteroinferior region of each hip bone. It supports the body when sitting. The pubis forms the anterior portion of the hip bone. The pubis curves medially, where it joins to the pubis of the opposite hip bone at a specialized joint called the pubic symphysis.
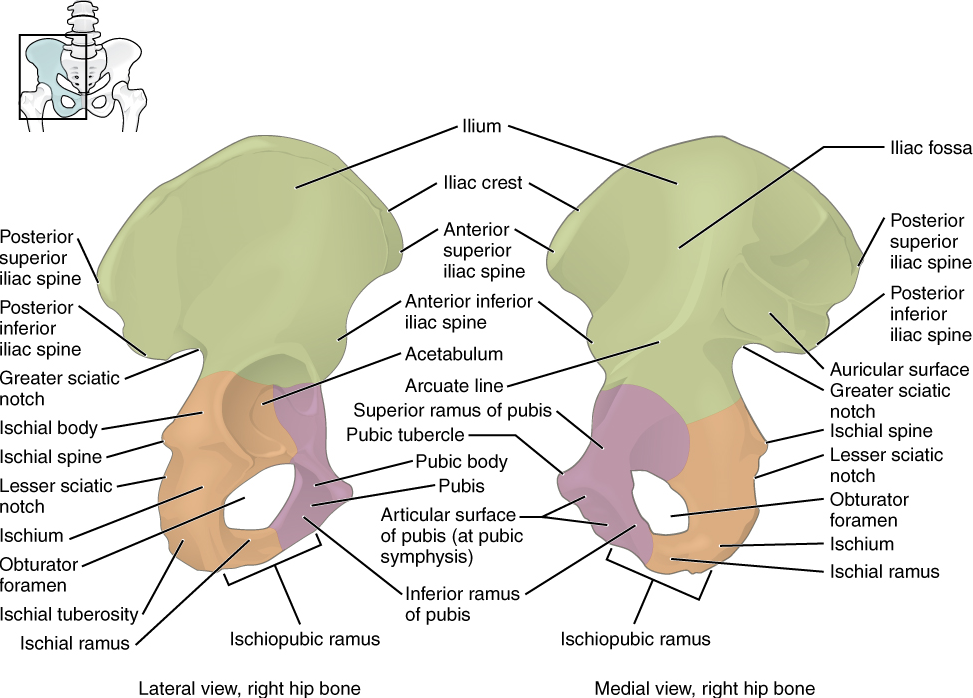
Pelvis: The pelvis consists of four bones: the right and left hip bones, the sacrum, and the coccyx (Figure 10.36). The pelvis has several important functions. Its primary role is to support the weight of the upper body when sitting and to transfer this weight to the lower limbs when standing. It serves as an attachment point for trunk and lower limb muscles and also protects the internal pelvic organs.
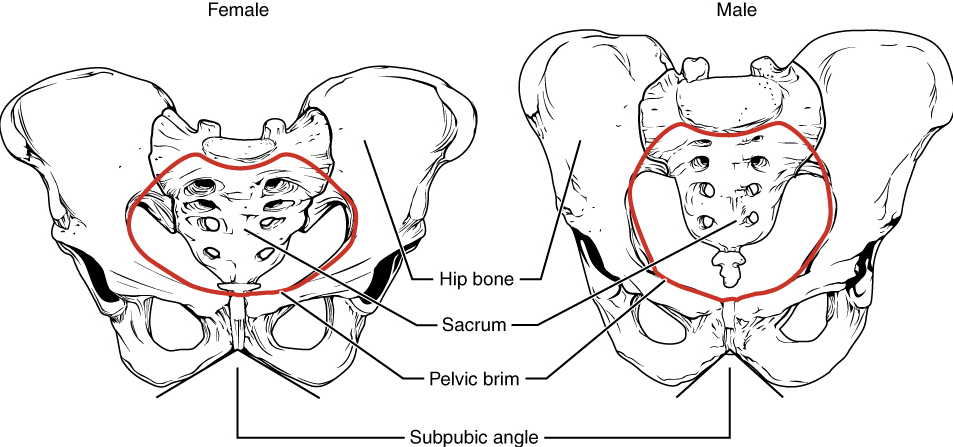
Comparison of the Female and Male Pelvis: The differences between the adult female and male pelvis relate to function and body size. In general, the bones of the male pelvis are thicker and heavier, adapted for support of the male’s heavier physical build and stronger muscles; this average size difference is generally true of other bones of the skeleton as well. The pelvis does show more robust differences between males and females due to its functional relationship to bipedal movement (requiring a relatively narrow pelvis) and birth of infants with large brains (requiring a relatively broad pelvis). Because the female pelvis is adapted for childbirth, it is wider than the male pelvis, as evidenced by the distance between the anterior superior iliac spines (Figure 10.38). The ischial tuberosities of females are also farther apart, which increases the size of the pelvic outlet. Because of this increased pelvic width, the subpubic angle is larger in females (greater than 80 degrees) than it is in males (less than 70 degrees). The female sacrum is wider, shorter, and less curved, and the sacral promontory projects less into the pelvic cavity, thus giving the female pelvic inlet (pelvic brim) a more rounded or oval shape compared to males. The pelvic cavity of females is also wider and shallower than the narrower, deeper, and tapering lesser pelvis of males. The greater sciatic notch of the male hip bone is narrower and deeper than the broader notch of females. Because of the obvious differences between female and male hip bones, this is the one bone of the body that allows for the most accurate sex determination. Table 10.4 provides an overview of the general differences between the female and male pelvis.
Female pelvis | Male pelvis | |
---|---|---|
Pelvic weight | Bones are lighter and thinner | Bones are thicker and heavier |
Pelvis inlet shape | Round or oval | Heart-shaped |
Lesser pelvic cavity shape | Shorter and wider | Longer and narrower |
Subpubic angle | Greater than 80 degrees | Less than 70 degrees |
Pelvic outlet shape | Rounded and larger | Smaller |
Test Your Knowledge
I. Describe the differences between an average male pelvis and an average female pelvis.
II. Why are male and female pelves generally shaped differently? For each sex difference noted in the question above, briefly explain the functional relevance of that sex difference specifically.
Part 4: Bones of the Lower Limb
Like the upper limb, the lower limb is divided into three regions. The thigh is the portion of the lower limb located between the hip joint and knee joint. The leg is specifically the region between the knee joint and the ankle joint. Distal to the ankle is the foot. The lower limb contains 30 bones. These are the femur, patella, tibia, fibula, tarsal bones, metatarsal bones, and phalanges (Figure 10.29). The femur is the single bone of the thigh. The patella is the kneecap and articulates with the distal femur. The tibia is the larger, weight-bearing bone located on the medial side of the leg, and the fibula is the thin bone of the lateral leg. The bones of the foot are divided into three groups. The posterior portion of the foot is formed by a group of seven bones, each of which is known as a tarsal bone, whereas the mid-foot contains five elongated bones, each of which is a metatarsal bone. The toes contain 14 small bones, each of which is a phalanx bone of the foot.
Femur: The femur, or thigh bone, is the single bone of the thigh region (Figure 10.39). It is the longest and strongest bone of the body and accounts for approximately one-quarter of a person’s total height. The rounded, proximal end is the head of the femur, which articulates with the acetabulum of the hip bone to form the hip joint.
Patella: The patella (kneecap) is the largest sesamoid bone of the body (see Figure 10.39). A sesamoid bone is a bone that is incorporated into the tendon of a muscle where that tendon crosses a joint. The sesamoid bone articulates with the underlying bones to prevent damage to the muscle tendon due to rubbing against the bones during movements of the joint. The patella is found in the tendon of the quadriceps femoris muscle, the large muscle of the anterior thigh that passes across the anterior knee to attach to the tibia. The patella articulates with the patellar surface of the femur and thus prevents rubbing of the muscle tendon against the distal femur. The patella also lifts the tendon away from the knee joint, which increases the leverage power of the quadriceps femoris muscle as it acts across the knee. The patella does not articulate with the tibia.
Tibia: The tibia (shin bone) is the medial bone of the leg and is larger than the fibula, with which it is paired (Figure 10.40). The tibia is the main weight-bearing bone of the lower leg and the second longest bone of the body, after the femur. The medial side of the tibia is located immediately under the skin, allowing it to be easily palpated down the entire length of the medial leg.
Fibula: The fibula is the slender bone located on the lateral side of the leg (Figure 10.40). The fibula does not bear weight. It serves primarily for muscle attachments and thus is largely surrounded by muscles. Only the proximal and distal ends of the fibula can be palpated.
Tarsal Bones: The posterior half of the foot is formed by seven tarsal bones (Figure 10.43). The most superior tarsal bone, the talus, articulates with the tibia and fibula to form the ankle joint. Inferiorly, the talus articulates with the calcaneus (heel bone), the largest bone of the foot, which forms the heel. Body weight is transferred from the tibia to the talus to the calcaneus, which rests on the ground.
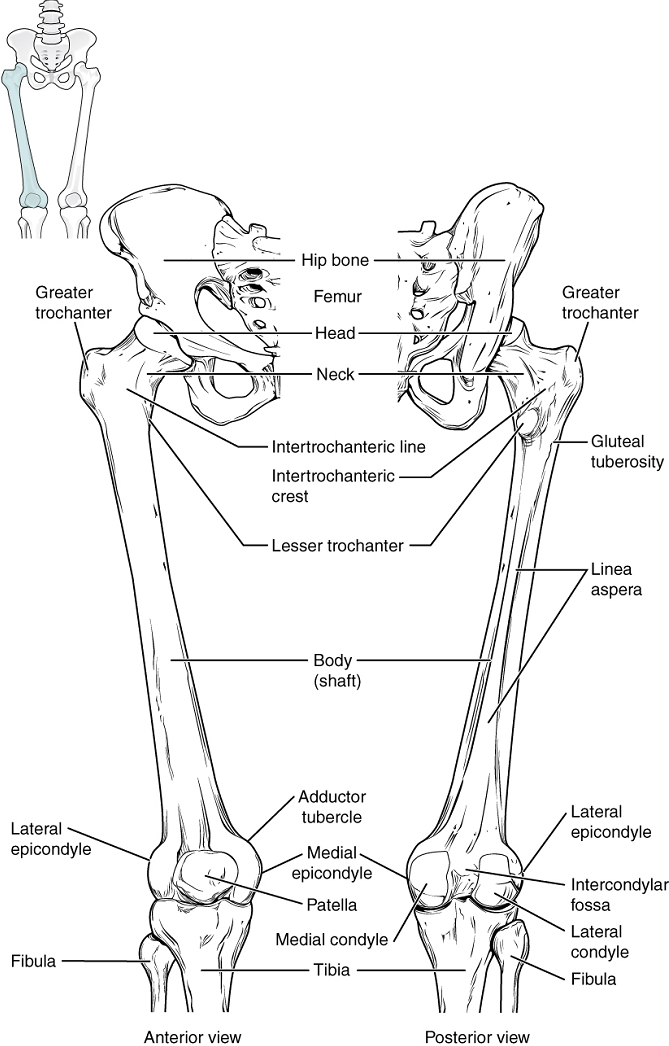
Metatarsal Bones: The anterior half of the foot is formed by the five metatarsal bones, which are located between the tarsal bones of the posterior foot and the phalanges of the toes (Figure 10.41). These elongated bones are numbered 1–5, starting with the medial side of the foot.
Phalanx bones: The toes contain a total of 14 phalanx bones (phalanges), arranged in a similar manner as the phalanges of the fingers (Figure 10.41). The toes are numbered 1–5, starting with the big toe (hallux). The big toe has two phalanx bones, the proximal and distal phalanges. The remaining toes all have proximal, middle, and distal phalanges. A joint between adjacent phalanx bones is called an interphalangeal joint.
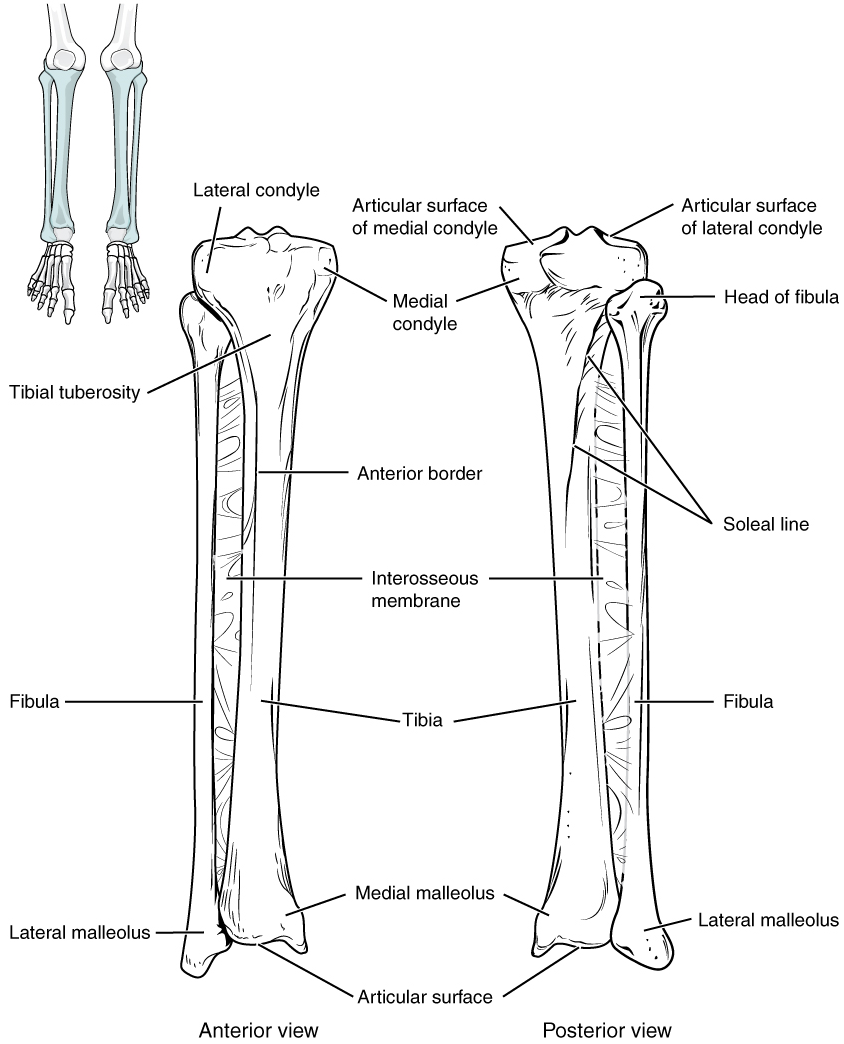
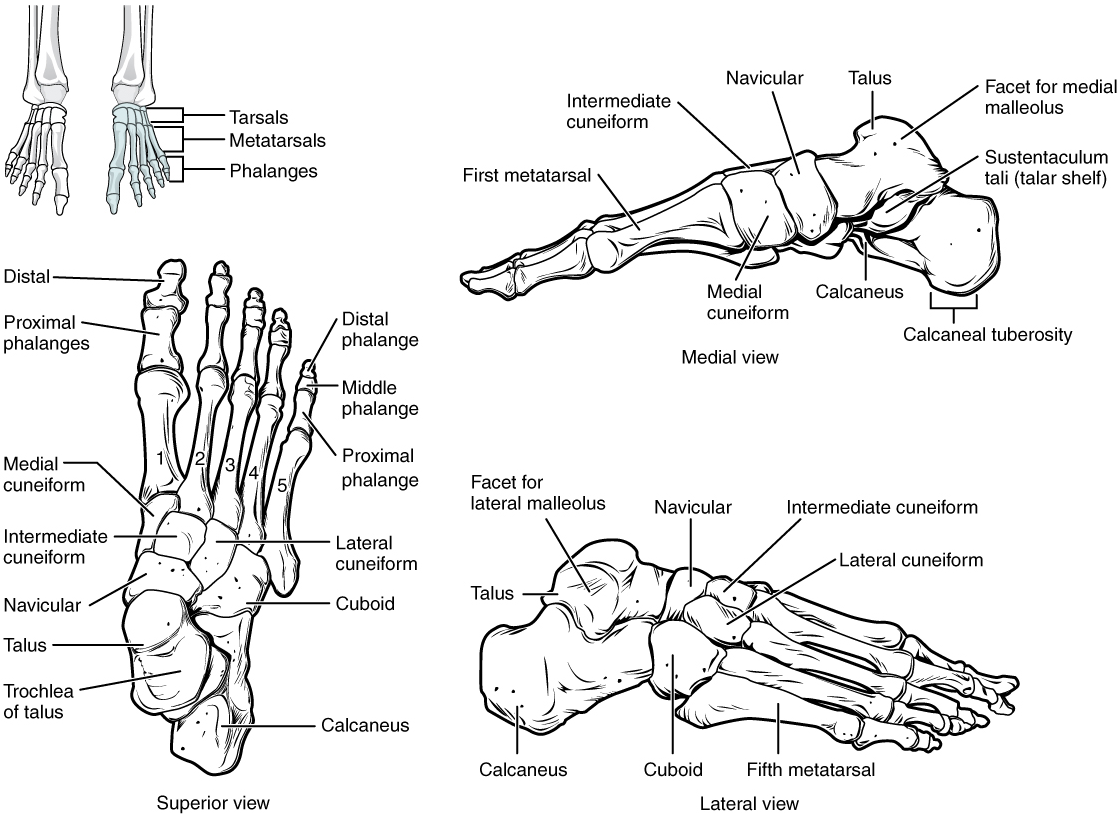
Test Your Knowledge
I. Identify and describe the bones of the lower limbs.
II. Identify the three groups of the foot bones.
Practice
For the activity below, drag the structure names to the correct empty boxes on the figure.
Image Descriptions
Tube composed of cartilaginous rings and supporting tissue that connects the lung bronchi and the larynx; provides a route for air to enter and exit the lung.
cartilaginous structure that produces the voice, prevents food and beverages from entering the trachea, and regulates the volume of air that enters and leaves the lungs.
Strong connective tissue bands that hold the bones at a moveable joint together.
Dense regular connective tissue that attaches skeletal muscle to bone.
Simple machine consisting of a beam or rigid rod (bone) pivoted at a fixed hinge, or fulcrum (joint).
Site at which two or more bones or bone and cartilage come together (articulate).
Fluid or semi-fluid portion of the matrix.
Connective tissue in the interior cavity of a bone where fat is stored.
Specialized areolar tissue rich in stored fat.
Connective tissue in the interior cavity of a bone where hematopoiesis takes place.
(Also, hematopoiesis) production of the formed elements of blood.
(Also, thrombocytes) one of the formed elements of blood that consists of cell fragments broken off from megakaryocytes.
Single bone of the upper arm.
Bone located on the medial side of the forearm.
Bone located on the lateral side of the forearm.
Thigh bone; the single bone of the thigh.
Shin bone; the large, weight-bearing bone located on the medial side of the leg.
Thin, non-weight-bearing bone found on the lateral side of the leg.
One of the five long bones that form the palm of the hand; numbered 1–5, starting on the lateral (thumb) side of the hand.
One of the five elongated bones that forms the anterior half of the foot; numbered 1–5, starting on the medial side of the foot.
(Plural = phalanges) one of the bones that forms the fingers or toes.
One of the eight small bones that form the wrist and base of the hand; these are grouped as a proximal row consisting of (from lateral to medial) the scaphoid, lunate, triquetrum, and pisiform bones, and a distal row containing (from lateral to medial) the trapezium, trapezoid, capitate, and hamate bones.
One of the seven bones that make up the posterior foot; includes the calcaneus, talus, navicular, cuboid, medial cuneiform, intermediate cuneiform, and lateral cuneiform bones.
Shoulder blade bone located on the posterior side of the shoulder.
Flattened bone located at the center of the anterior chest.
Knee cap.
Tubular shaft that runs between the proximal and distal ends of a long bone.
Wide section at each end of a long bone; filled with spongy bone and red marrow.
Hollow region of the diaphysis; filled with yellow marrow.
Dense osseous tissue that can withstand compressive forces.
(Also, cancellous bone) trabeculated osseous tissue that supports shifts in weight distribution.
(Also, growth plate) sheet of hyaline cartilage in the metaphysis of an immature bone; replaced by bone tissue as the organ grows in length.
Most common type of cartilage, smooth and made of short collagen fibers embedded in a chondroitin sulfate ground substance.
completely ossified remnant of the epiphyseal plate
Delicate membranous lining of a bone’s medullary cavity.
Fibrous membrane covering the outer surface of bone and continuous with ligaments.
Thin layer of cartilage covering an epiphysis; reduces friction and acts as a shock absorber.
Layer of spongy bone that is sandwiched between two the layers of compact bone found in flat bones.
The most abundant of three protein fibres found in the extracellular matrix of connective tissues.
A form of calcium phosphate mineral found in bones (also hydroxylapatite).
Cell responsible for forming new bone.
Primary cell in mature bone; responsible for maintaining the matrix.
(Plural = lacunae) small spaces in bone or cartilage tissue that cells occupy.
(Singular = canaliculus) channels within the bone matrix that house one of an osteocyte’s many cytoplasmic extensions that it uses to communicate and receive nutrients.
Division of genetic material, during which the cell nucleus breaks down and two new, fully functional, nuclei are formed. Usually immediately followed by cytokinesis (cell division).
Undifferentiated cell with high mitotic activity; the only bone cells that divide; they differentiate and develop into osteoblasts.
Cell responsible for resorbing bone.
Precursor to macrophages and dendritic cells seen in the blood.
Ameboid (irregular outline with peripheral projections) phagocyte found in several tissues throughout the body.
(Also, Haversian system) basic structural unit of compact bone; made of concentric layers of calcified matrix.
Concentric rings of calcified matrix that form an osteon.
(Also Haversian canal) longitudinal channel in the center of each osteon; contains blood vessels, nerves, and lymphatic vessels.
(Also, Volkmann’s canal) channel that branches off from the central canal and houses vessels and nerves that extend to the periosteum and endosteum.
(Singular= trabecula) spikes or sections of the lattice-like matrix in spongy bone.
Small opening in the middle of the external surface of the diaphysis, through which an artery enters the bone to provide nourishment.
(Also, osteogenesis) bone formation.
Process by which bone forms directly from mesenchymal tissue.
Process in which bone forms by replacing hyaline cartilage.
Cell responsible for forming new cartilage.
Lacking blood vessels.
Embryonic tissue from which connective tissue cells derive.
Collarbone; elongated bone that articulates with the manubrium of the sternum medially and the acromion of the scapula laterally.
Cluster of osteoblasts found in the early stages of intramembranous ossification.
Uncalcified bone matrix secreted by osteoblasts.
Region, deep in the periosteal collar, where bone development starts during endochondral ossification.
Type of cartilaginous joint where the bones are joined by fibrocartilage.
Layer of dense irregular connective tissue surrounding cartilage.
cartilage cells
Region of bone development in the epiphyses.
Region of the epiphyseal plate that anchors the plate to the osseous tissue of the epiphysis.
Region of the epiphyseal plate that makes new chondrocytes to replace those that die at the diaphyseal end of the plate and contributes to longitudinal growth of the epiphyseal plate.
Region of the epiphyseal plate where chondrocytes from the proliferative zone grow and mature and contribute to the longitudinal growth of the epiphyseal plate.
Region of the epiphyseal plate closest to the diaphyseal end; functions to connect the epiphyseal plate to the diaphysis.
(In connective tissue) extracellular material that is produced by the cells embedded in it, containing ground substance and fibers.
Central, vertical axis of the body, including the skull, vertebral column, and thoracic cage.
Entire sequence of bones that extend from the skull to the tailbone.
Consists of 12 pairs of ribs and sternum.
Small, U-shaped bone located in upper neck that does not contact any other bone.
Three small bones in the middle ear.
Individual bone in the neck and back regions of the vertebral column.
Single bone located near the inferior end of the adult vertebral column that is formed by the fusion of five sacral vertebrae; forms the posterior portion of the pelvis.
Lowest part of the vertebral column; 'tailbone'
Describes the front or direction toward the front of the body; also referred to as ventral.
Fourteen bones that support the facial structures and form the upper and lower jaws and the hard palate.
Unpaired bone that forms the lower jaw bone; the only moveable bone of the skull.
Expanded area of fibrous connective tissue that separates the braincase bones of the skull prior to birth and during the first year after birth.
Fibrous joint that connects the bones of the skull (except the mandible); an immobile joint (synarthrosis).
Division of the posterior (dorsal) cavity that houses the brain.
Paired bones that form the upper, lateral sides of the skull.
Paired bones that form the lateral, inferior portions of the skull, with squamous, mastoid, and petrous portions.
Unpaired bone that forms forehead, roof of orbit, and floor of anterior cranial fossa.
Unpaired bone that forms the posterior portions of the braincase and base of the skull.
Unpaired bone that forms the central base of skull.
Unpaired bone that forms the roof and upper, lateral walls of the nasal cavity, portions of the floor of the anterior cranial fossa and medial wall of orbit, and the upper portion of the nasal septum.
Paired bones that form the lateral, inferior portions of the skull, with squamous, mastoid, and petrous portions.
(Also, maxilla) paired bones that form the upper jaw and anterior portion of the hard palate.
Structure located between the bodies of adjacent vertebrae that strongly joins the vertebrae; provides padding, weight bearing ability, and enables vertebral column movements.
anterior portion of each vertebra that supports the body weight.
Bony arch formed by the posterior portion of each vertebra that surrounds and protects the spinal cord.
Opening associated with each vertebra defined by the vertebral arch that provides passage for the spinal cord.
Bony passageway within the vertebral column for the spinal cord that is formed by the series of individual vertebral foramina.
General anatomical term for a hole or opening (usually in bone. Plural = foramina).
Paired bony processes that extends laterally from the vertebral arch of a vertebra.
Unpaired bony process that extends posteriorly from the vertebral arch of a vertebra.
Bony process that extends upward from the vertebral arch of a vertebra that articulates with the inferior articular process of the next higher vertebra.
Bony process that extends downward from the vertebral arch of a vertebra that articulates with the superior articular process of the next lower vertebra.
Paired bony processes that extends laterally from the vertebral arch of a vertebra.
First cervical (C1) vertebra.
Second cervical (C2) vertebra.
Bony projection (odontoid process) that extends upward from the body of the C2 (axis) vertebra.
Expanded, superior portion of the sternum.
Small process that forms the inferior tip of the sternum.
Hyaline cartilage structure attached to the anterior end of each rib that provides for either direct or indirect attachment of most ribs to the sternum.
Vertebrosternal ribs 1–7 that attach via their costal cartilage directly to the sternum.
Vertebrochondral ribs 8–12 whose costal cartilage either attaches indirectly to the sternum via the costal cartilage of the next higher rib or does not attach to the sternum at all.
Vertebral ribs 11–12 that do not attach to the sternum or to the costal cartilage of another rib.
All bones of the upper and lower limbs, plus the girdle bones that attach each limb to the axial skeleton.
An encircling or confining structure; in anatomy, the pectoral or pelvic girdle.
Shoulder girdle; the set of bones, consisting of the scapula and clavicle, which attaches each upper limb to the axial skeleton.
Hip girdle; consists of a single hip bone, which attaches a lower limb to the sacrum of the axial skeleton.
Describes the middle or direction toward the middle of the body.
Describes the back or direction toward the back of the body; also referred to as dorsal.
Describes the side or direction toward the side of the body.
(Also, glenoid fossa) shallow depression located on the lateral scapula, between the superior and lateral borders.
Shoulder joint; formed by the articulation between the glenoid cavity of the scapula and the head of the humerus.
Describes a position in a limb that is nearer to the point of attachment or the trunk of the body.
Wrist joint, located between the forearm and hand regions of the upper limb; articulation formed proximally by the distal end of the radius and the fibrocartilaginous pad that unites the distal radius and ulna bone and distally by the scaphoid, lunate, and triquetrum carpal bones.
Describes a position in a limb that is farther from the point of attachment or the trunk of the body.
Thumb
Articulation between adjacent phalanx bones of the hand or foot digits.
Superior portion of the hip bone.
Superior portion of the hip bone.
Anterior portion of the hip bone.
Joint formed by the articulation between the auricular surfaces of the sacrum and ilium.
Joint formed by the articulation between the pubic bodies of the right and left hip bones.
Large, roughened protuberance that forms the posteroinferior portion of the hip bone; weight-bearing region of the pelvis when sitting.
Large, U-shaped indentation located on the posterior margin of the ilium, superior to the ischial spine.
A bone embedded in tendon; the only major sesamoid bone is the patella.
Muscle deep to the gluteus maximus on the lateral surface of the thigh that laterally rotates the femur at the hip.
Tarsal bone that articulates superiorly with the tibia and fibula at the ankle joint; also articulates inferiorly with the calcaneus bone and anteriorly with the navicular bone.
Heel bone; posterior, inferior tarsal bone that forms the heel of the foot.
Big toe