Chapter 2. Homeostasis
Sharon Lagarde
Unit Outline
Learning Objectives
At the end of this unit, you should be able to:
I. Explain the importance of homeostasis to physiology and specify three conditions that are maintained by homeostatic processes.
II. Describe the internal environment of the human body.
III. Define the term stressor.
IV. Define a feedback loop. Explain what is meant by negative and positive feedback systems and describe their role in homeostasis.
Part 1: Homeostasis
Homeostasis refers to a relatively stable set of conditions within an organism’s internal environment. Within the human body, maintaining a healthy environment for living cells requires maintaining appropriate conditions in the extracellular fluids—including the interstitial fluid and blood plasma—for each living cell to be able to function properly. The constancy of an internal environment is important in allowing chemical reactions to take place at rates necessary to support the body.
Test Your Knowledge
- Write a definition of homeostasis.
- State the three conditions that are maintained by homeostasis, and for each, describe at least one consequence to human physiology if that condition deviates from the normal range.
Conditions Maintained by Homeostasis
There are three important conditions that must be met in order for the chemical reactions in the body to occur at the rates necessary for homeostasis. The first condition is that there must be a proper concentration of gases, nutrients, water, and salts. The gases involved are oxygen, which is necessary for the process of cellular respiration to produce energy for the body, and carbon dioxide, which is a waste product of this process but also must be present in certain amounts for other processes to occur efficiently. Nutrients provide reactants, enzymes, cofactors, and energy for chemical reactions, while water and salts determine the fluid balance, electrolyte balance, and the pH of the body.
The second important condition for homeostasis is an optimum temperature that is around 37°C. The rates of chemical reactions are temperature dependent, typically occurring slower at cooler temperatures and faster at warmer temperatures. Additionally, the shapes of proteins (remember that proteins fulfill many roles in the body, including providing structure, muscle contraction, and enzymatic function, among others) are temperature dependent—if they overheat, they may denature and be rendered non-functional.
The third and final condition is an optimum pressure, for example, blood pressure. The concentrations of various substances in the body and the rates at which these substances move through the body are both dependent on blood pressure. Blood pressure is influenced by the volume of blood in the cardiovascular system, the diameter of the blood vessels and the strength of cardiac muscle contraction.
Test Your Knowledge
- Write a definition of homeostasis.
- State the three conditions that are maintained by homeostasis, and for each, describe at least one consequence to human physiology if that condition deviates from the normal range.
The Internal Environment
Homeostasis is maintained within the internal environment of the body. The human body is composed of cells surrounded by extracellular material, which is mostly fluid. Cells are responsible for controlling bodily activities and also controlling the composition of the environment around them. The internal environment is defined as the fluid inside the body but outside of cells.
The fluid inside the cells in the body is the intracellular fluid, also called cytoplasm (cytosol and the fluid inside organelles). Intracellular fluid makes up approximately 2/3 of the total body fluids.
The fluid outside the cells in the body is the extracellular fluid and is synonymous with the internal environment. Extracellular fluid is found in blood (i.e. plasma), in tissues (i.e. interstitial fluid), in the lymphatic system (i.e., lymph), in joints (i.e., synovial fluid), in the eyes (i.e., aqueous and vitreous humors), and in the central nervous system (i.e., cerebrospinal fluid). Extracellular fluid amounts to about 1/3 of the fluids in the body as a whole.
The three main fluid types within the extracellular compartment are plasma, interstitial fluid and lymph. Plasma is the liquid portion of blood and functions to move red and white blood cells, platelets, nutrient molecules, gases, electrolytes, and wastes throughout the circulatory system. Materials are exchanged at capillary beds where the blood vessel walls are thin enough to allow materials to move between the blood and the tissues.
The fluid that surrounds living cells within tissues is called interstitial fluid, which has a unique composition relative to both intracellular fluid and blood plasma. Components of plasma move into the tissues, thus the interstitial fluid is partly derived from the plasma of blood. Interstitial fluid is also in contact with cells and is thus partly derived from the intracellular fluid.
Interstitial fluid must be returned to the circulatory system in order to maintain appropriate pressure within the cardiovascular system and for waste removal from cells. However, the interstitial fluid is under lower pressure than the plasma in the capillaries, and so only 85% of the interstitial fluid is returned directly back to the circulatory system at the capillaries (mostly as a result of the osmotic gradient; the higher concentration of solutes inside the capillaries “sucks” the water back in). The remaining 15% is returned indirectly through the vessels of another system called the lymphatic system. The fluid in these vessels, now called lymph, is derived from tissue fluid but is different from it in composition. Lymph is collected into larger lymphatic vessels that eventually drain into veins close to the heart.
Although the extracellular and intracellular compartments are distinct from one another, there is a continual exchange of materials between them. Cells must receive nutrients, have waste materials taken away, and exchange gases. Refer to Chapter 7, Cell Biology: Membrane Transport, to review how substances move between intracellular and extracellular compartments.
Test Your Knowledge
- Define internal environment.
- Draw an annotated diagram (a diagram with explanatory labels) showing extracellular fluid, intracellular fluid, plasma, interstitial fluid, and lymph. Be sure to include a label that defines the internal environment.
- On your diagram, add annotations to describe how substances move between fluid compartments.
Stressors
A constant internal environment is impossible to maintain. The goal of homeostasis is to ensure that the constant changes to the internal environment are kept within a stable range. The internal environment is continually subjected to disturbances that, unless counteracted, would quickly cause a new set of conditions in the body that could result in illness or death. A stressor is any stimulus that causes an imbalance in the internal environment. Stressors may be factors external to the body or from within the body itself.
An example of an external stressors is a lack of environmental oxygen, which may occur at high altitudes or extreme environmental temperatures. Examples of internal stressors are rapid changes in blood pressure—hemorrhaging can cause a drop in pressure, while unpleasant thoughts may raise blood pressure—or changes in levels of nutrients, such as an increase or decrease in blood glucose levels.
Test Your Knowledge
- Write a definition of a stressor.
- Describe two examples of internal stressors (originate within the body).
- Describe two examples of external stressors (originate from the external environment).
Part 2: Feedback loops
Maintaining homeostasis requires that the body continuously monitor its internal conditions. From body temperature to blood pressure to nutrient levels, each physiological condition has a particular set point. A set point is the physiological value around which the normal range fluctuates. A normal range is the restricted set of values that is optimally healthy and stable. For example, the set point for normal human body temperature is approximately 37°C (98.6°F); however, physiological body temperature tends to fluctuate within a few degrees above and below that set point depending on factors such as activity, digestive function, stress, etc. Control centers in the brain and other parts of the body monitor and react to deviations from homeostasis using negative feedback. Negative feedback is a mechanism that reverses a deviation from the set point. Therefore, negative feedback maintains body parameters within their normal range. The maintenance of homeostasis by negative feedback occurs throughout the body at all times, and an understanding of negative feedback is thus fundamental to an understanding of human physiology.
Negative Feedback
Feedback systems have five basic components (Figure 2.1). A sensor, also referred to a receptor, detects when the stressor/stimulus produces a deviation in a physiological value away from the set point. This change in value is reported to the control center. The control center is the component in a feedback system that compares the value to the normal range. If the value deviates too much from the set point, then the control center activates an effector. An effector is the component in a feedback system that produces a response, which in the case of a negative feedback loop, causes a return of the physiological value to its normal range.
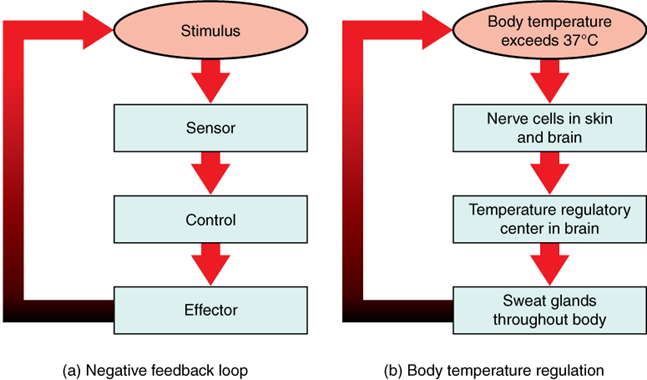
For example, in the control of blood glucose, specific endocrine cells (receptors) in the pancreas detect excess glucose (stimulus) in the bloodstream. These pancreatic beta cells (control center) respond to the increased level of blood glucose by releasing the hormone insulin into the bloodstream. The insulin signals skeletal muscle fibers, fat cells (adipocytes), and liver cells (effectors) to take up the excess glucose (response), removing it from the bloodstream. As the glucose concentration in the bloodstream drops, the decrease in concentration—the actual negative feedback (outcome)—is detected by pancreatic beta cells, and insulin release stops. This helps prevent blood sugar levels from continuing to drop below the normal range.
Humans have a similar temperature regulation feedback system that works by promoting either heat loss or heat gain (Figure 2.1b). When the brain’s temperature regulation center in the hypothalamus receives data from the sensors indicating that the body’s temperature exceeds its normal range, it stimulates a cluster of brain cells referred to as the thermoregulatory center. This stimulation has three major effects:
- Blood vessels in the skin begin to dilate, allowing more blood from the body core to flow to the surface of the skin and allowing heat to radiate into the external environment.
- As blood flow to the skin increases, sweat glands are activated to increase their output. As sweat evaporates from the skin surface into the surrounding air, it lowers the temperature of the skin’s surface.
- The depth of respiration increases, and a person may breathe through an open mouth instead of through the nasal passageways. This further increases heat loss from the lungs.
In contrast, activation of the thermoregulatory center by exposure to cold reduces blood flow to the skin, and blood returning from the limbs is diverted into a network of deep veins. This arrangement traps heat closer to the body core and restricts heat loss. If heat loss is severe, the hypothalamus triggers an increase in random signals to skeletal muscles, causing them to contract and producing the shivering response. The muscle contractions involved in shivering release heat while using ATP. The brain triggers the thyroid gland in the endocrine system to release the thyroid hormone, which increases metabolic activity and heat production in cells throughout the body. The hypothalamus also signals the adrenal glands to release epinephrine (adrenaline), a hormone that causes the breakdown of glycogen into glucose, which can be used as an energy source. The breakdown of glycogen into glucose also results in increased metabolism and heat production.
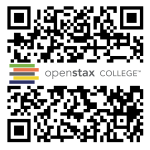
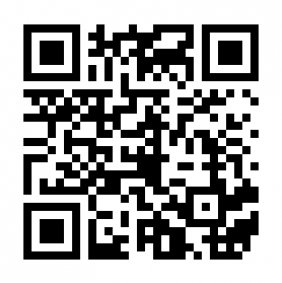
Positive Feedback
Positive feedback intensifies a change in the body’s physiological condition rather than reversing it. Positive feedback loops employ the same five basic components as negative feedback loops, with the difference being that a deviation from the normal range results in more change, and the system moves farther away from the set point. Positive feedback in the body is much more rare than negative feedback and often occurs when a dramatic change in normal physiological activity is desired. Childbirth, blood clotting, and micturition are some examples of physiological positive feedback loops (there are a number of other examples in immunity, in female reproductive cycles and at the level of cellular function).
Childbirth at full term is an example of a situation in which the maintenance of the existing body state is not desired. Enormous changes in the mother’s body are required to expel the baby at the end of pregnancy. And the events of childbirth, once begun, must progress rapidly to a conclusion, or the life of the mother and the baby are at risk. The extreme muscular work of labor and delivery are the result of a positive feedback system (Figure 2.2).
The first contractions of labor (the stimulus) push the baby toward the cervix (the lowest part of the uterus). The cervix contains stretch-sensitive nerve cells that monitor the degree of stretching (the sensors). These nerve cells send messages to the brain, which in turn causes the pituitary gland at the base of the brain (the control center) to release the hormone oxytocin into the bloodstream. Oxytocin causes stronger contractions of involuntary smooth muscles in the uterus (the effectors), pushing the baby farther down the birth canal. This causes even greater stretching of the cervix (the response). The cycle of stretching, oxytocin release, and increasingly more forceful contractions stops only when the baby is born. At this point, the stretching of the cervix halts, stopping the release of oxytocin.
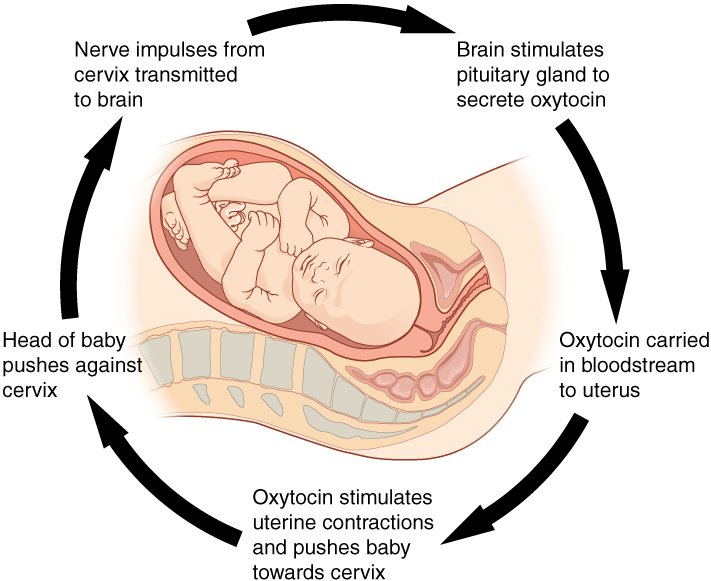
A second example of positive feedback centers on reversing extreme damage to the body. Following a penetration wound, the most immediate threat is excessive blood loss. Less blood circulating means reduced blood pressure and reduced perfusion (distribution of blood) to the brain and other vital organs. If perfusion is severely reduced, vital organs will shut down, and the person will die. The body responds to this potential catastrophe by releasing substances in the injured blood vessel wall that begin the process of blood clotting. As each step of clotting occurs, it stimulates the release of more clotting substances. This accelerates the processes of clotting and seals off the damaged area. Clotting is contained in a local area based on the tightly controlled availability of clotting proteins. This is an adaptive, life-saving cascade of events.
Test Your Knowledge
- Define a feedback loop. Explain what is meant by negative and positive feedback systems and describe their role in homeostasis.
- Write definitions for set point and normal range as they pertain to homeostasis.
- Draw a flowchart to describe a generalized negative feedback loop including all of the following components: stimulus, receptor, control center, effector, response. Annotate your flowchart with a description of the role of each of these components in the functioning of a feedback loop.
- Use appropriate terminology to clearly distinguish between positive feedback mechanisms and negative feedback mechanisms. (Important: do NOT use “shorthand” descriptions for this! Make sure you use appropriate and precise terminology and complete sentences.)
- Use all the terms from question 2, above, along with the specific body structures representing each (where appropriate), to describe in detail:
- One physiological negative feedback mechanism used in the human body.
- One physiological positive feedback mechanism used in the human body.
- Positive feedback mechanisms are likely to continue out of control and are often associated with disease states. Using your example of a physiological positive feedback mechanism from the previous question, explain how the positive feedback loop is shut down once the function is served.
Practice
For the following questions, click the correct answer choice.
Image Descriptions
Figure 2.1 Negative Feedback Loop. A negative feedback loop is a normal biological response in which the effects of a reaction slow or stop that reaction. A negative feedback loop helps regulate health by ensuring that a reaction is appropriate and that the systems of the body are in a constant state of equilibrium, also known as homeostasis. When a system falls out of homeostasis, your body will react with hormones, enzymes, and other substances to correct this imbalance. A negative feedback loop effectively “puts the brakes” on the reaction once homeostasis is achieved and systems are stabilized. [Return to image.]
Figure 2.2 Positive Feedback Loop. Childbirth is an example of a positive feedback loop. The first contractions of labor (the stimulus) push the baby toward the cervix (the lowest part of the uterus). The cervix contains stretch-sensitive nerve cells that monitor the degree of stretching (the sensors). These nerve cells send messages to the brain, which in turn causes the pituitary gland at the base of the brain to release the hormone oxytocin into the bloodstream. Oxytocin causes stronger contractions of the smooth muscles of the uterus (the effectors), pushing the baby farther down the birth canal. This causes even greater stretching of the cervix. The cycle of stretching, oxytocin release and increasingly more forceful contractions stops only when the baby is born. [Return to image.]
Fluid outside cells (plasma or interstitial fluid).
Extracellular fluid in the small spaces between cells not contained within blood vessels.
An extracellular fluid, the fluid component of blood.
Production of ATP from glucose oxidation via glycolysis, the Krebs cycle, and oxidative phosphorylation.
Molecule (usually a protein) that catalyzes chemical reactions.
Cofactors are inorganic substances that bind to enhance enzyme function; dietary minerals, such as zinc, often play the role of cofactors.
A solution containing ions; sometimes referring to ions themselves.
Fluid inside cells.
Fluid contained within the lymphatic system, consisting of interstitial fluid, leukocytes (white blood cells), proteins (including antibodies), and fats.
Group of many similar cells (though sometimes composed of a few related types) that work together to perform a specific function.
(In physiology) any stimulus that causes an imbalance in the internal environment.
Steady state of body systems that living organisms maintain.
(In physiology) ideal value for a physiological parameter; the level or small range within which a physiological parameter such as blood pressure is stable and optimally healthful, that is, within its parameters of homeostasis.
Homeostatic mechanism that tends to stabilize an upset in the body’s physiological condition by preventing an excessive response to a stimulus, typically as the stimulus is removed.
Protein molecule that contains a binding site for another specific molecule (called a ligand).
Compares values to their normal range; deviations cause the activation of an effector.
Organ that can cause a change in a value.
Tissue or organ that secretes hormones into the blood and lymph without ducts such that they may be transported to organs distant from the site of secretion.
Secretion of an endocrine organ that travels via the bloodstream or lymphatics to induce a response in target cells or tissues in another part of the body.
Lipid storage cells.
Region of the brain inferior to the thalamus that functions in neural and endocrine signaling, temperature regulation, and control of the autonomic nervous system.
Becoming wider, larger, or more open.
Mechanism that intensifies a change in the body’s physiological condition in response to a stimulus.
Also called urination or voiding.