Chapter 3. Atoms and Molecules
Sharon Lagarde
Unit Outline
Learning Objectives
At the end of this unit, you should be able to:
I. Define the term “chemical element,” specify the name and symbol for the four most common chemical elements in the body, and describe the importance of each.
II. Define the term “atom” and describe the structure of an atom.
III. Define the terms “molecule” and “compound.”
IV. Describe the formation of an ion and of an ionic bond.
V. Describe the formation of a covalent bond.
VI. Distinguish between organic and inorganic molecules.
VII. Describe the composition of organic molecules, specify two characteristics of organic molecules that make them useful to living organisms, and give examples of organic molecules.
VIII. Specify the chemical properties of water.
The smallest, most fundamental material components of the human body are chemical elements. All of the elements found in the human body—elements that include phosphorus, carbon, sodium, and calcium, to name a few—originated in stars. These elements, in turn, form both the inorganic and organic chemical compounds that are important to life, including, for example, water, glucose, and proteins. This chapter will begin by examining elements and how the structures of atoms, the basic units of matter, determine the characteristics of elements by the number of protons, neutrons, and electrons in the atoms.
Part 1: Elements and Atoms: The Building Blocks of Matter
Every substance of the universe—from a grain of sand to a star—is called matter. Matter is defined as anything that occupies space and has mass.
Elements and Compounds
All matter in the natural world is composed of one or more fundamental substances called elements. An element is a pure substance that is distinguished from all other matter by the fact that it cannot be created or broken down by ordinary chemical means. While your body can assemble many of the chemical compounds needed for life from their constituent elements, it cannot make elements. They must come from the environment.
The elements in the human body are shown in Figure 3.1, beginning with the four most abundant: oxygen (O), carbon (C), hydrogen (H), and nitrogen (N). Each element’s name can be replaced by a one- or two-letter symbol; you will become familiar with some of these throughout this course. All the elements in your body are derived from the foods you eat and the air you breathe.
In nature, elements rarely occur alone. The combination of two or more atoms joined by chemical bonds is called a molecule. A compound is a substance containing at least two different elements joined by chemical bonds.
All compounds can be categorized based on the nature of their constituent elements. An inorganic compound is a substance that does not contain both carbon and hydrogen. A great many inorganic compounds do contain hydrogen atoms, such as water (H2O) and the hydrochloric acid (HCl) produced by your stomach. In contrast, only a handful of inorganic compounds contain carbon atoms. Carbon dioxide (CO2) is one of the few examples.
An organic compound, then, is a substance that contains carbon-hydrogen bonds. Many organic compounds can be synthesized via covalent bonds within the human body. Recall that carbon and hydrogen are the second and third most abundant elements in your body. You will soon discover how these two elements combine in the foods you eat, in the compounds that make up your body structure, and in the chemicals that fuel your daily activities.
Carbon atoms can bind to other carbon atoms as well as atoms of other elements in multiple ways; so organic molecules come in many different shapes with different properties depending on their exact chemical composition. The fact that small organic molecules can be assembled into very large molecules with complex structures makes them useful to living cells in several ways. Examples include structural components of cells or functional components that allow chemical reactions to proceed.
Finally, the chemical energy present in the covalent bonds in many organic molecules can be transferred to other molecules in the form of new bonds. For example, some of the energy contained in the bonds of a glucose molecule can be harvested and used by living cells to attach a phosphate group to a molecule of adenosine diphosphate (ADP), converting the molecule to adenosine triphosphate (ATP). The vital importance of ATP to the functioning of human cells is discussed in more detail throughout this textbook.
The compound glucose is an important body fuel. It is composed of carbon, hydrogen, and oxygen. Because it is a carbon-based molecule that contains hydrogen, it is an organic compound. The elements that make up any given compound always occur in the same relative amounts. In glucose, there are always six carbon units and six oxygen units for every twelve hydrogen units. But what, exactly, are these “units” of elements?
Test Your Knowledge
- Define the term “chemical element.”
- For each of the four most common chemical elements in the human body:
-
- Specify its name
- Specify its chemical symbol
- Name at least one molecule or type of molecule in which it is found, and briefly describe that molecule’s function in the human body.
- Describe the composition of organic molecules, specify two characteristics of organic molecules that make them useful to living organisms, and give examples of organic molecules.
- Compare and contrast (list similarities and differences between) organic molecules and inorganic molecules.
- Name at least two specific examples of each type of molecule (i.e., name two organic molecules and two inorganic molecules).
- Describe the chemical composition of organic molecules.
- Name the four main types of organic molecules discussed further in this course. (Hint: you may need to look at the “Biochemistry” topic to answer this question!)
- Specify at least two characteristics of organic molecules that make them particularly useful to living organisms.
Atoms and Subatomic Particles
An atom is the smallest quantity of an element that retains the unique properties of that element. In other words, an atom of hydrogen is a unit of hydrogen—the smallest amount of hydrogen that can possibly exist. As you might guess, atoms are almost unfathomably small. The period at the end of this sentence is millions of atoms wide.
1. Atomic Structure and Energy: Atoms are made up of even smaller subatomic particles, three types of which are important: the proton, neutron, and electron. The number of positively charged protons and non-charged (“neutral”) neutrons determines the mass of an atom, and the number of protons in the nucleus of the atom determines the element. The number of negatively charged electrons equals the number of protons, giving all elements on the periodic table a neutral charge.
There are different ways to illustrate the structure of an atom (Figure 3.2). Consider a typical atom of helium (He), which is composed of two protons, two neutrons, and two electrons. In the planetary model (or Bohr model), helium’s two electrons are shown circling the nucleus in a fixed orbit depicted as a ring (Figure 3.2a). Although this model is helpful in visualizing atomic structure, in reality, electrons do not travel in fixed orbits but whiz around the nucleus erratically in a so-called electron cloud (Figure 3.1).
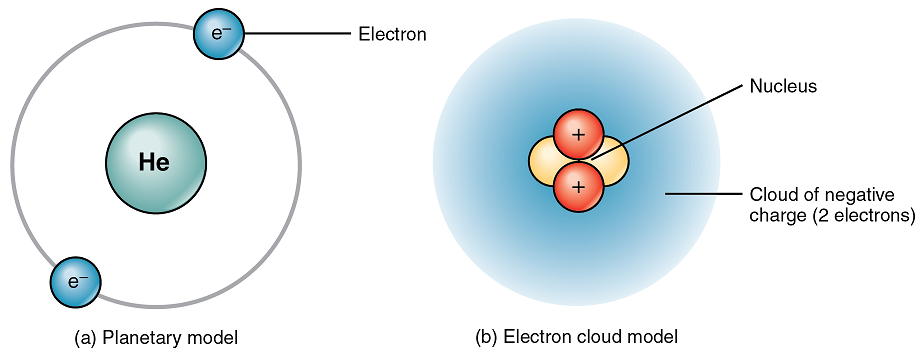
An atom’s protons and electrons carry electrical charges. An atom’s neutrons have no charge: they are electrically neutral. Just as a magnet sticks to a steel refrigerator because their opposite charges attract, the positively charged protons attract the negatively charged electrons. This mutual attraction gives the atom some structural stability. The attraction to the positively charged nucleus keeps electrons from straying away.
2. Atomic Number and Mass Number: An atom of carbon is unique to carbon, but a proton of carbon is not. One proton is the same as another, whether it is found in an atom of carbon, sodium (Na), or iron (Fe). The same is true for neutrons and electrons. So what gives an element its distinctive properties—what makes carbon so different from sodium or iron? The answer is the unique quantity of protons each contains. Carbon, by definition, is an atom whose nucleus contains six protons. No other element has exactly six protons in its nucleus. Moreover, all atoms of carbon, whether found in your liver or in a lump of coal, contain six protons. Thus, the atomic number, which is the number of protons in the nucleus of the atom, identifies the element. Because elements have the same number of electrons as protons, the atomic number identifies the number of electrons as well.
In their most common form, many elements also contain the same number of neutrons as protons. The most common form of carbon, for example, has six neutrons and six protons for a total of 12 subatomic particles in its nucleus. An element’s mass number is the sum of the number of protons and neutrons in its nucleus. So the most common mass number of carbon is 12. (Electrons have so little mass that they do not appreciably contribute to the mass of an atom.) Carbon is a relatively light element. Uranium (U), in contrast, has a mass number of 238 and is referred to as a heavy metal. Its atomic number is 92 (it has 92 protons), but it contains 146 neutrons and has the most mass of all the naturally occurring elements.
The periodic table of the elements (see Appendix I) is a chart identifying the 92 elements found in nature, as well as several larger, unstable elements discovered experimentally. The elements are arranged in order of their atomic number, with hydrogen and helium at the top of the table and the more massive elements below. The periodic table is a useful device because for each element, it identifies the chemical symbol, the atomic number, and the mass number while organizing elements according to their propensity to react with other elements. The number of protons and electrons in an element are equal. The number of protons and neutrons may be equal for some elements but are not equal for all.
3. The Behavior of Electrons: In the human body, atoms do not exist as independent entities. Rather, they are constantly reacting with other atoms to form and break down more complex substances. To fully understand anatomy and physiology, you must grasp how atoms participate in such reactions. The key is understanding the behavior of electrons.
Although electrons do not follow rigid orbits a set distance away from the atom’s nucleus, they do tend to stay within certain regions of space called electron shells. An electron shell is a layer of electrons that encircle the nucleus at a distinct energy level (Figure 3.2).
The elements found in the human body have from one to five electron shells; the first shell holds up to two, the second shell holds up to eight, the third shell holds up to eighteen. All electron shells apart from the first shell may be considered “complete” with eight electrons, thus making the atom non-reactive. This configuration of electron shells is the same for all atoms. The precise number of shells depends on the number of electrons in the atom. Hydrogen and helium have just one and two electrons, respectively. If you take a look at the periodic table of the elements, you will notice that hydrogen and helium are placed alone on either side of the top row; they are the only elements that have just one electron shell. A second shell is necessary to hold the electrons in all elements larger than hydrogen and helium.
Lithium (Li), whose atomic number is 3, has three electrons. Two of these electrons fill the first electron shell, and the third electron is found in the second shell. The second electron shell can accommodate as many as eight electrons. Carbon, with its six electrons, entirely fills its first shell and half-fills its second. With ten electrons, neon (Ne) entirely fills its two electron shells. A look at the periodic table reveals that all of the elements in the second row, from lithium to neon, have just two electron shells. Atoms with more than ten electrons require more than two shells. These elements occupy the third and subsequent rows of the periodic table.
The factor that most strongly governs the tendency of an atom to participate in chemical reactions is the number of electrons in its valence shell. A valence shell is an atom’s outermost electron shell. If the valence shell is full, the atom is stable; meaning its electrons are unlikely to be pulled away from the nucleus by the electrical charge of other atoms. Elements with full valence shells are described as inert, or non-reactive. If the valence shell is not full, the atom is reactive; meaning it will tend to react with other atoms in an attempt to fill its valence shell. Consider hydrogen, with its one electron only half-filling its valence shell. This single electron is likely to participate in the formation of chemical bonds with the other elements, so that hydrogen’s single valence shell can be stabilized.
All atoms (except hydrogen and helium with their single electron shells) are most stable when there are exactly eight electrons in their valence shell. This principle is referred to as the octet rule; and it states that an atom will give up, gain, or share electrons with another atom so that it ends up with eight electrons in its own valence shell. For example, oxygen, with six electrons in its valence shell, is likely to react with other atoms in a way that results in the addition of two electrons to oxygen’s valence shell, bringing the total to eight. When two hydrogen atoms each share their single electron with oxygen, covalent bonds are formed, resulting in the formation of a molecule of water, H2O.
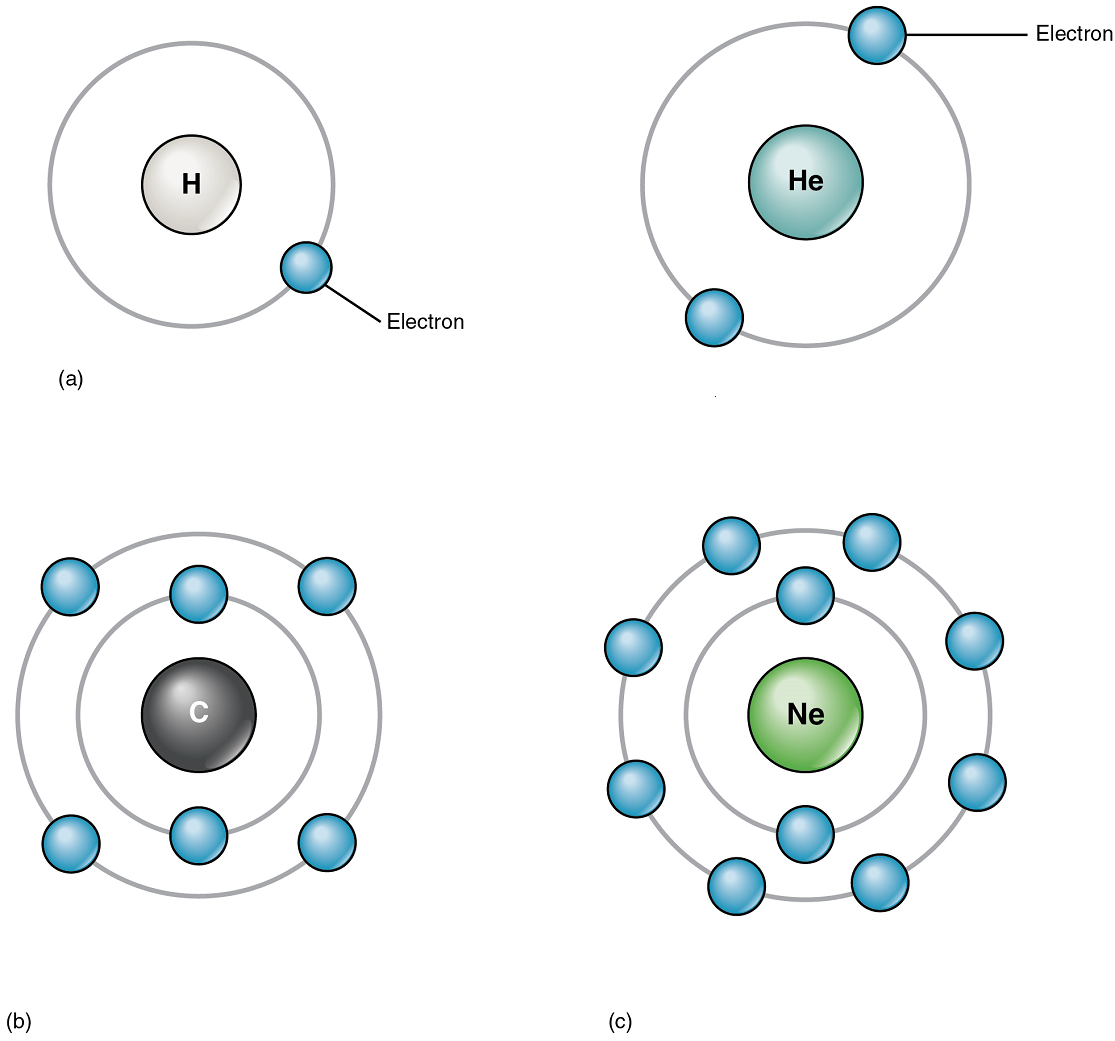
Test Your Knowledge
- Define the term “atom.”
- Draw an annotated diagram (a diagram that includes descriptive labels, with verbs) of the “planetary” (or “Bohr”) model describing the general structure of an atom.
- Be able to identify the number of electrons, protons and neutrons for common elements if given the atomic number and mass number.
- Define the terms “molecule” and “compound.”
- Write 1–2 sentences to clearly distinguish between the terms “molecule” and “compound.” (If a question ever asks you to “clearly distinguish between,” “compare,” “contrast,” or similar terms, make sure the direct comparison is very obvious in your answer!)
Part 2: Chemical Bonds
Ions and Ionic Bonds
Recall that an element has the same number of positively charged protons and negatively charged electrons. As long as this is the case, each atom of that element is electrically neutral. But when an atom participates in a chemical reaction that results in the donation or acceptance of one or more electrons, the atom will then become positively or negatively charged. This happens frequently for most atoms in order to have a full valence shell, as described previously. This can happen either by gaining electrons to fill a shell that is more than half-full or by giving away electrons to empty a shell that is less than half-full, thereby leaving the next smaller electron shell as the new, full valence shell. An atom that has an electrical charge—whether positive or negative—is an ion.
Potassium (K), for instance, is an important element in all body cells. Its atomic number is 19. It has just one electron in its valence shell. This characteristic makes potassium highly likely to participate in chemical reactions in which it donates one electron. (It is easier for potassium to donate one electron than to gain seven electrons.) The loss will cause the positive charge of potassium’s protons to be more influential than the negative charge of potassium’s electrons. In other words, the resulting potassium ion will be positive. A potassium ion is written as K+, indicating that it has lost a single electron and is now a positively charged ion known as a cation.
Now consider fluorine (F), a vital component of bones and teeth. Its atomic number is nine, and it has seven electrons in its valence shell. Thus, it is highly likely to bond with other atoms in such a way that fluorine accepts one electron (it is easier for fluorine to gain one electron than to donate seven electrons). When it does, its electrons will outnumber its protons by one, and it will have an overall negative charge. The ionized form of fluorine is called fluoride and is written as F−. A negatively charged ion is known as an anion.
Atoms that have more than one electron to donate or accept will end up with stronger positive or negative charges. A cation that has donated two electrons has a net charge of +2. Using magnesium (Mg) as an example, this can be written as Mg++ or Mg2+. An anion that has accepted two electrons has a net charge of −2. The ionic form of selenium (Se), for example, is typically written Se2−.
The opposite charges of cations and anions exert a moderately strong mutual attraction that keeps the atoms in close proximity, forming an ionic bond. An ionic bond is an ongoing, close association between ions of opposing charges. The table salt you sprinkle on your food owes its existence to ionic bonding (Figure 3.4). Sodium commonly donates an electron to chlorine, becoming the cation Na+. When chlorine accepts this electron, it becomes the chloride anion, Cl−. With their opposing charges, these two ions strongly attract each other. The molecules formed through ionic bonding are referred to as compounds.
One of the reasons that water is an essential component of life is because of its ability to break the ionic bonds in salts. In fact, in biological fluids, most individual atoms exist as ions. These dissolved ions produce electrical charges within the body. The behavior of these ions allows us to monitor heart and brain function observed as waves on an electrocardiogram (EKG or ECG) or an electroencephalogram (EEG). The electrical activity that derives from the interactions of these ions is why they are also called electrolytes.
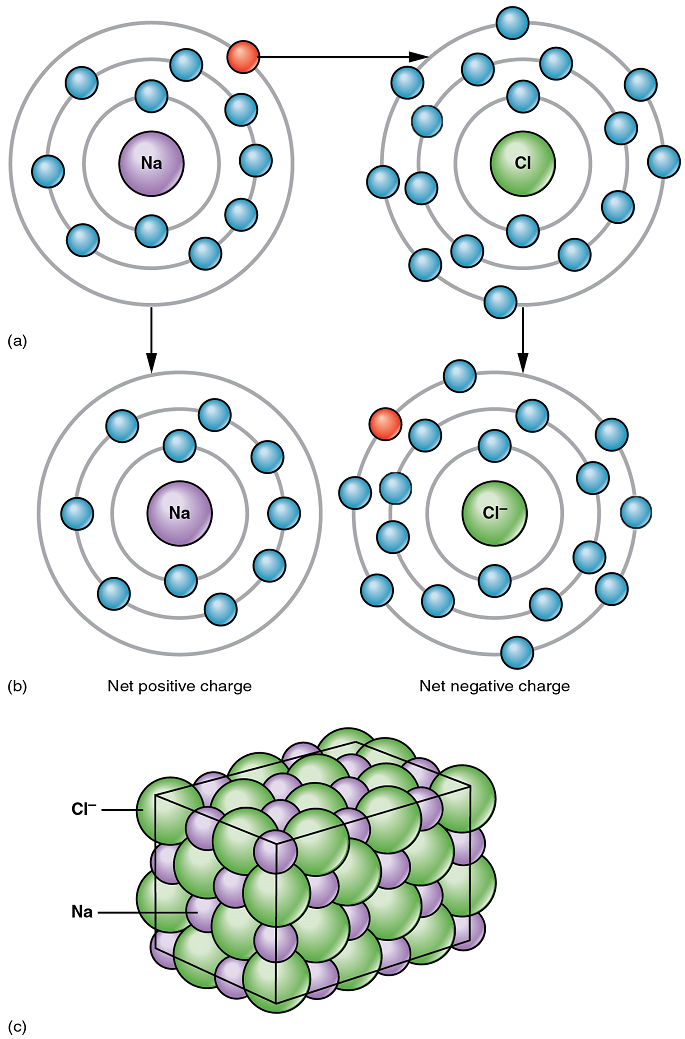
Test Your Knowledge
- Use an annotated diagram to describe the process by which ions are formed from neutral atoms.
- Write one sentence that states what is meant by the term “ionic bond.”
Covalent Bonds
Unlike ionic bonds formed by the attraction between a cation’s positive charge and an anion’s negative charge, molecules formed by a covalent bond share electrons in a mutually stabilizing relationship. Like next-door neighbors whose kids hang out first at one home and then at the other, the atoms do not lose or gain electrons permanently. Instead, the electrons move back and forth between the elements. Because of the close sharing of pairs of electrons (one electron from each of two atoms), most covalent bonds are not broken apart in water.
1. Nonpolar Covalent Bonds: Figure 3.4 shows several common types of covalent bonds. Notice that the two covalently bonded atoms typically share just one or two electron pairs, though larger sharings are possible. The important concept to take from this is that in covalent bonds, electrons in the outermost valence shell are shared to fill the valence shells of both atoms, ultimately stabilizing both of the atoms involved. In a single covalent bond, a single electron pair is shared between two atoms, while in a double covalent bond, two pairs of electrons are shared between two atoms. There even are triple covalent bonds, where three pairs of electrons are shared. You can see that the covalent bonds shown in Figure 3.4 are balanced. The sharing of the negative electrons is relatively equal, as is the electrical pull of the positive protons in the nucleus of the atoms involved. This is why covalently bonded molecules that are electrically balanced in this way are described as nonpolar; that is, no region of the molecule is either more positive or more negative than any other.
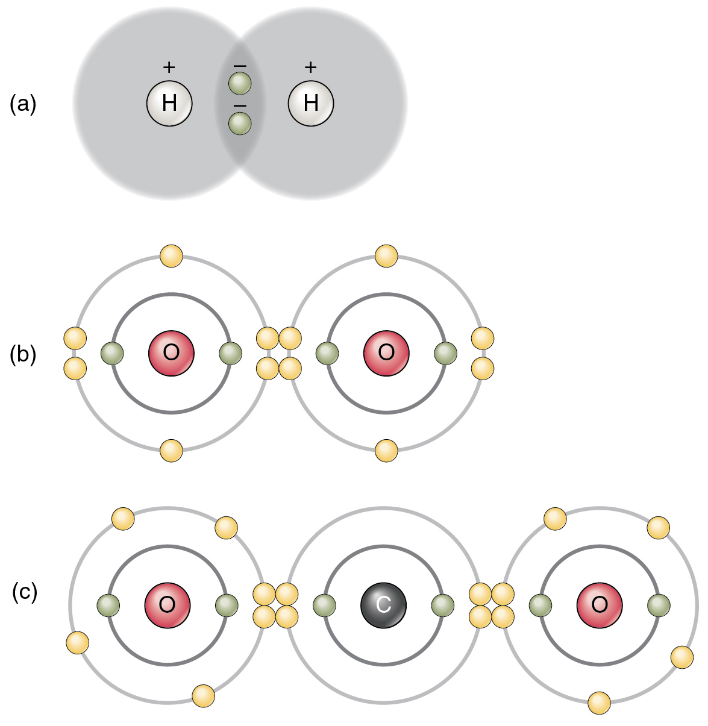
2. Polar Covalent Bonds: Politicians with completely opposite views on a particular issue are often described as “polarized” by the media. In chemistry, a polar molecule is a molecule that contains regions that have opposite electrical charges. Polar molecules occur when atoms share electrons unequally due to polar covalent bonds.
The most familiar example of a polar molecule is water (Figure 3.5). The molecule has three atoms: one atom of oxygen, the nucleus of which contains eight protons, and two hydrogen atoms, whose nuclei each contain only one proton. Because every proton exerts an identical positive charge, a nucleus that contains eight protons exerts a positive charge eight times greater than a nucleus that contains one proton. This means that the negatively charged electrons present in the water molecule are more strongly attracted to the oxygen nucleus than to the hydrogen nuclei. Each hydrogen atom’s single electron therefore migrates toward the oxygen atom, making the oxygen end of the bond slightly more negative than the hydrogen end of the bond.
What is true for the bonds is true for the water molecule as a whole; that is, the oxygen region has a slightly negative charge, and the regions of the hydrogen atoms have a slightly positive charge. These slight charges are also referred to as “partial charges” because the strength of the charge is less than one full electron, as would occur in an ionic bond. Regions of weak polarity are indicated in diagrams with the Greek letter delta (∂) and a plus (+) or minus (–) sign (Figure 3.4).
Even though a single water molecule is unimaginably tiny, it has mass, and the opposing electrical charges on the molecule pull that mass in such a way that it creates a somewhat triangular shape (Figure 3.5). The result is a dipole molecule with two distinct poles or regions of concentrated charge. The positive pole is formed by two small hydrogen atoms at the “bottom” of the triangle, while the negative pole is formed by a single large oxygen atom at the “top” of the triangle).
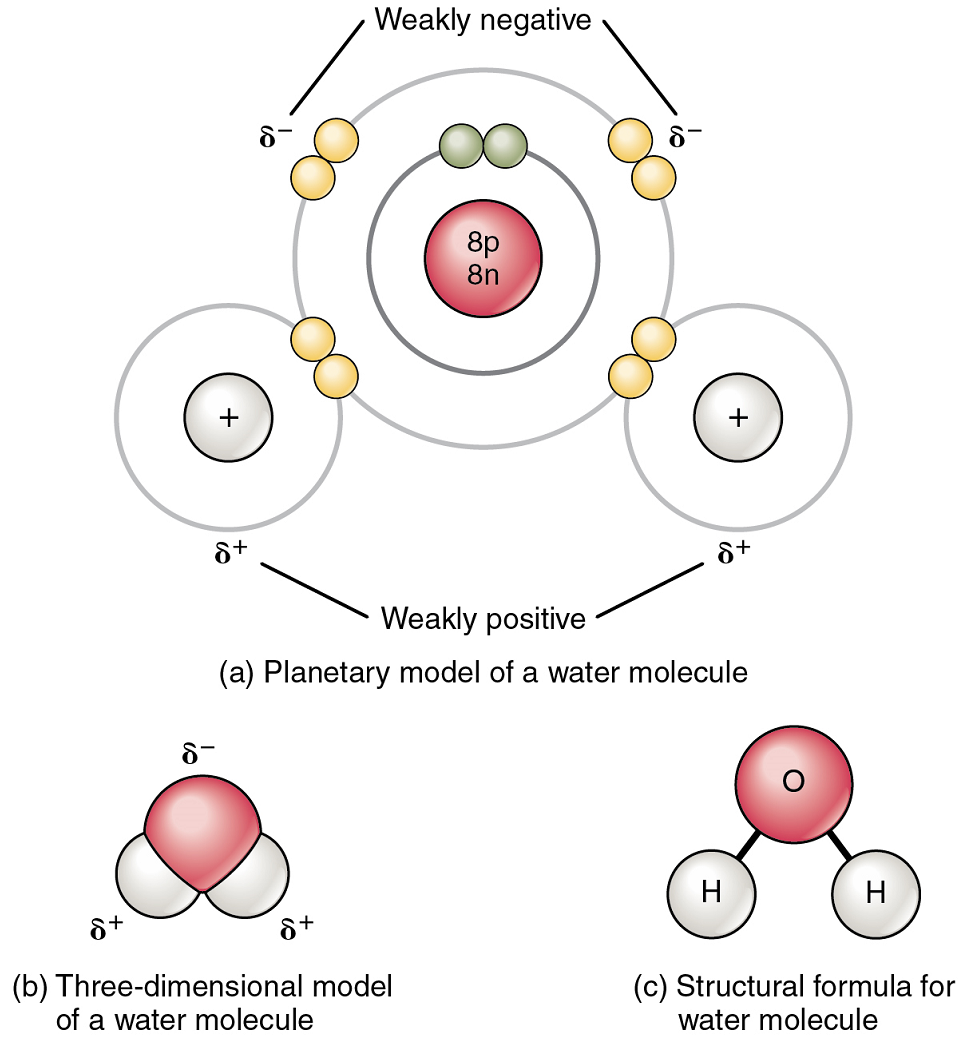
Test Your Knowledge
- Describe the process by which a covalent bond is formed between two atoms.
- Compare and contrast (list similarities and differences between) ionic compounds and covalent molecules.
Hydrogen Bonds
A hydrogen bond is formed when a slightly (or weakly) positive hydrogen atom is already bonded to one electronegative atom (for example, the oxygen in the water molecule) and is attracted to another electronegative atom from another molecule. In other words, hydrogen bonds always include a hydrogen atom that is already part of a polar molecule.
The most common example of hydrogen bonding in the natural world occurs between separate molecules of water. It happens before your eyes whenever two raindrops merge into a larger bead, or a creek spills into a river. Hydrogen bonding occurs because the slightly negative oxygen atom in one water molecule is attracted to the slightly positive hydrogen atoms of two other water molecules (Figure 3.6).
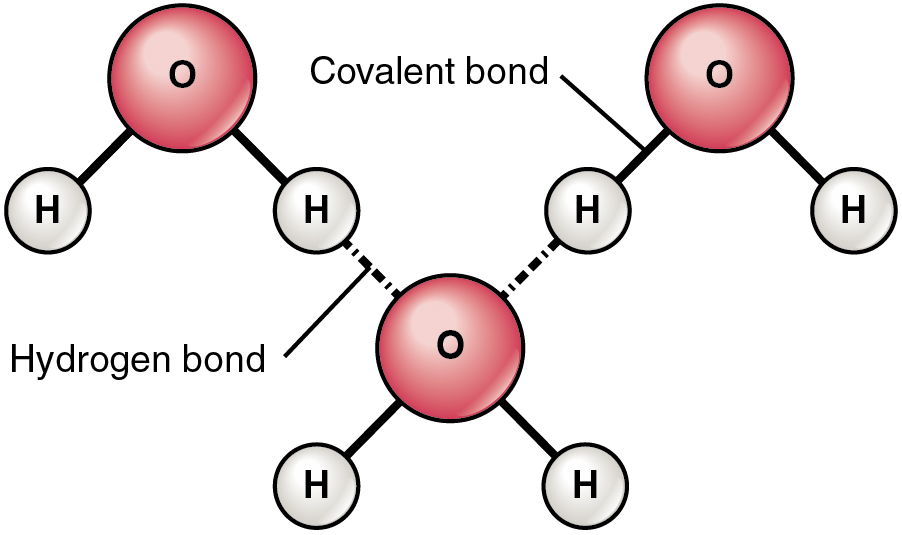
Water molecules strongly attract all charged molecules, including ions. This explains why sodium chloride or “table salt,” for example, which consists of equal numbers of positively charged sodium (Na+) and negatively charged chloride (Cl−) atoms, dissolves so readily in water. In this case, dipole–ion bonds form between the water and the electrically charged ions (electrolytes), allowing moving water molecules to pull the Na+ and Cl− away from each other. Water molecules also repel molecules with non-polar covalent bonds, like fats, lipids, and oils. You can demonstrate this with a simple kitchen experiment: pour a teaspoon of vegetable oil, a compound formed by non-polar covalent bonds, into a glass of water. Instead of instantly dissolving in the water, the oil forms a distinct bead because the polar water molecules repel the non-polar oil.
Physical Properties of Water
The formation of hydrogen bonds in water allows water to have different physical properties than other liquids. One is density. For a typical substance, the solid state is denser than the liquid state. However, this is not the case for water, as ice (solid state) is actually less dense than water (liquid state). The formation of hydrogen bonds in water also affects its boiling point. Scientific predictions based on chemical trends indicate that water should have a boiling point of −90⁰C. However, the actual boiling point of water is 100⁰C. In a similar manner, hydrogen bonding also affects the specific heat capacity of water so that water requires an unusually high amount of energy be added to increase its temperature (and releases an unusually high amount of energy when cooled). These unique properties allow water to very effectively moderate human body temperature.
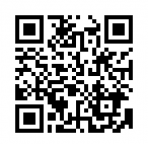
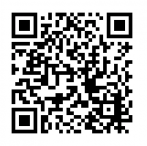
Test Your Knowledge
- Draw a diagram of a single water molecule. Clearly label any:
- Oxygen atoms
- Hydrogen atoms
- Covalent bonds
- Draw an annotated diagram showing two, and only two, water molecules. In your diagram, you should include all the labels shown above, then add explanatory labels identifying and fully describing the following important chemical properties:
- The molecule’s polarity
- Its molecular shape
- The important interaction (“bond”) that exists between two water molecules
- Describe how the chemical properties of water affects the physical properties of water.
Practice
For the following questions, click the correct answer choice
A substance that cannot be created or broken down by ordinary chemical means.
A substance that does not contain both carbon and hydrogen.
A substance that contains both carbon and hydrogen.
Monosaccharide commonly used as energy in the body (substrate for glycolysis).
Class of organic compounds that are composed of many amino acids linked together by peptide bonds.
The smallest unit of an element that retains the unique properties of that element.
A heavy subatomic particle having a positive charge and found in the atom’s nucleus.
A heavy subatomic particle having no electrical charge and found in the atom’s nucleus.
A subatomic particle having a negative charge and nearly no mass; found orbiting the atom’s nucleus.
Scientifically, a physical substance; that which occupies space and has mass
A substance composed of two or more different elements joined by chemical bonds.
Two or more atoms covalently bonded together.
Chemical bond in which two atoms share electrons, thereby completing their valence shells.
Lower energy form of ATP, containing two phosphate groups after the third phosphate group phosphorylated another molecule and transferring energy to it.
Nucleotide containing ribose and an adenine base that is essential in energy transfer.
Number of protons in the nucleus of an atom.
Sum of the number of protons and neutrons in the nucleus of an atom.
An arrangement of the elements in a table according to their atomic number; elements having similar properties because of their electron arrangements compose columns in the table, while elements having the same number of valence shells compose rows in the table. See Appendix I.
Area of space a given distance from an atom’s nucleus in which electrons are grouped.
Outermost electron shell of an atom.
Atom with an overall positive or negative charge. Many function as electrolytes.
Ion with a positive charge.
Atom with a negative charge.
Attraction between an anion and a cation.
Surface recording of the electrical activity of the heart that can be used for diagnosis of irregular heart function.
Chemical bond in which two atoms share electrons, thereby completing their valence shells.
Opposite of polar; molecule with electrons that are relatively equally shared in covalent bonds.
Molecule with regions that have opposite charges resulting from uneven numbers of electrons in the nuclei of the atoms participating in the covalent bond.
Molecule with a negatively charged portion separated from a positively charged portion.
Dipole-dipole bond in which a hydrogen atom covalently bonded to an electronegative atom is weakly attracted to a second electronegative atom.
The tendency of an atom to attract electrons in a covalent bond.
Mass per unit volume.