23
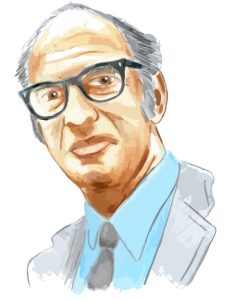
Biography of Thomas Kuhn
Thomas Kuhn, born on July 18, 1922, and passed away on June 17, 1996, was an influential philosopher of science known for his groundbreaking work on the nature and history of scientific revolutions. His ideas have had a profound impact on the field of science studies and have reshaped our understanding of how scientific knowledge progresses and undergoes transformative changes.
Kuhn earned his PhD in physics from Harvard University in 1949, but he later shifted his focus to the philosophy of science. His seminal work, The Structure of Scientific Revolutions, published in 1962, revolutionized the field and introduced the concept of paradigm shifts. Kuhn argued that scientific progress is not a steady accumulation of knowledge but rather occurs through revolutionary periods where dominant scientific paradigms are replaced by new ones.
According to Kuhn, scientific communities operate within specific conceptual frameworks called paradigms, which include shared theories, methods, and assumptions. These paradigms shape scientists’ perception of reality and guide their research efforts. However, when anomalies and inconsistencies arise that cannot be easily explained within the existing paradigm, a crisis may occur. This crisis leads to the exploration of new ideas and the emergence of alternative paradigms, eventually resulting in a scientific revolution.
Kuhn’s work challenged the traditional view of science as a purely objective and cumulative process and highlighted the social and subjective aspects of scientific inquiry. He emphasized the role of scientific communities, social norms, and historical context in shaping scientific knowledge and determining scientific progress.
The Structure of Scientific Revolutions had a profound impact on various fields, including philosophy, history, and sociology of science. Kuhn’s ideas sparked lively debates and influenced generations of scholars who sought to understand the complex dynamics of scientific change. His concept of paradigm shifts has become a foundational concept in science studies, and his work continues to shape contemporary discussions on the nature of scientific knowledge.
Throughout his career, Kuhn held academic positions at institutions such as the University of California, Berkeley, Princeton University, and the Massachusetts Institute of Technology. He received numerous accolades for his contributions, including the prestigious MacArthur Fellowship in 1982.
Thomas Kuhn’s intellectual legacy extends far beyond his own discipline, inspiring critical examinations of the history, sociology, and philosophy of science. His ideas have challenged traditional views of scientific progress and have provided a rich framework for understanding the complex interplay between theory, observation, and social factors in scientific development.
Vol. II, No. 2
44
The Priority of Paradigms
(Note: Materials are included on the basis of fair use as described in the Code of Best Practices for Fair Use in Open Education.)
What need we know, Wittgenstein asked, in order that we apply terms like ‘chair,’ or ‘leaf,’ or ‘game’ unequivocally and without provoking argument?2 That question is very old and has generally been answered by saying that we must know, consciously or intuitively, what a chair, or leaf, or game is. We must, that is, grasp some set of attributes that all games and that only games have in common. Wittgenstein, however, concluded that, given the way we use language and the sort of world to which we apply it, there need be no such set of characteristics. Though a discussion of some of the attributes shared by a number of games or chairs or leaves often helps us learn how to employ the corresponding term, there is no set of characteristics that is simultaneously applicable to all members of the class and to them alone. Instead, confronted with a previously unobserved activity, we apply the term ‘game’ because what we are seeing bears a close “family resemblance” to a number of the activities that we have previously learned to call by that name. For Wittgenstein, in short, games, and chairs, and leaves are natural families, each constituted by a network of overlapping and crisscross resemblances. The existence of such a network sufficiently accounts for our success in identifying the corresponding object or activity. Only if the families we named overlapped and merged gradually into one another—only, that is, if there were no natural families—would our success in identifying and naming provide evidence for a set of common characteristics corresponding to each of the class names we employ. Something of the same sort may very well hold for the various research problems and techniques that arise within a single normal scientific tradition. What these have in common is not that they satisfy some explicit or even some fully discoverable set of rules and assumptions that gives the tradition its character and its hold upon the scientific mind. Instead, they may relate by resemblance and by modeling to one or another part of the scientific corpus which the community in question already recognizes as among its established achievements.
Scientists work from models acquired through education and through subsequent exposure to the literature often without quite knowing or needing to know what characteristics have given these models the status of community paradigms. And because they do so, they need no full set of rules. The coherence displayed by the research tradition in which they participate may not imply even the existence of an underlying body of rules and assumptions that additional historical or philosophical investigation might uncover. That scientists do not usually ask or debate what makes a particular problem or solution legitimate tempts us to suppose that, at least intuitively, they know the answer. But it may only indicate that neither the question nor the answer is felt to be relevant to their research. Paradigms may be prior to, more binding, and more complete than any set of rules for research that could be unequivocally abstracted from them. So far this point has been entirely theoretical: paradigms could determine normal science without the intervention of discoverable rules. Let me now try to increase both its clarity and urgency by indicating some of the reasons for believing that paradigms actually do operate in this manner. The first, which has already been discussed quite fully, is the severe difficulty of discovering the rules that have guided particular normal-scientific traditions. That difficulty is very nearly the same as the one the philosopher encounters when he tries to say what all games have in common. The second, to which the first is really a corollary, is rooted in the nature of scientific education. Scientists, it should already be clear, never learn concepts, laws, and theories in the abstract and by themselves. Instead, these intellectual tools are from the start encountered in a historically and pedagogically prior unit that displays them with and through their applications. A new theory is always announced together with applications to some concrete range of natural phenomena; without them it would not be even a candidate for acceptance. After it has been accepted, those same applications or others accompany the theory into the textbooks from which the future practitioner will learn his trade. They are not there merely as embroidery or even as documentation. On the contrary, the process of learning a theory depends upon the study of applications, including practice problem-solving both with a pencil and paper and with instruments in the laboratory. If, for example, the student of Newtonian dynamics ever discovers the meaning of terms like ‘force,’ ‘mass,’ ‘space,’ and ‘time,’ he does so less from the incomplete though sometimes helpful definitions in his text than by observing and participating in the application of these concepts to problem-solution. That process of learning by finger exercise or by doing continues throughout the process of professional initiation. As the student proceeds from his freshman course to and through his doctoral dissertation, the problems assigned to him become more complex and less completely precedented. But they continue to be closely modeled on previous achievements as are the problems that normally occupy him during his subsequent independent scientific career. One is at liberty to suppose that somewhere along the way the scientist has intuitively abstracted rules of the game for himself, but there is little reason to believe it. Though many scientists talk easily and well about the particular individual hypotheses that underlie a concrete piece of current research, they are little better than laymen at characterizing the established bases of their field, its legitimate problems and methods. If they have learned such abstractions at all, they show it mainly through their ability to do successful research. That ability can, however, be understood without recourse to hypothetical rules of the game. These consequences of scientific education have a converse that provides a third reason to suppose that paradigms guide research by direct modeling as well as through abstracted rules. Normal science can proceed without rules only so long as the relevant scientific community accepts without question the particular problem-solutions already achieved. Rules should therefore become important and the characteristic unconcern about them should vanish whenever paradigms or models are felt to be insecure. That is, moreover, exactly what does occur. The pre-paradigm period, in particular, is regularly marked by frequent and deep debates over legitimate methods, problems, and standards of solution, though these serve rather to define schools than to produce agreement. We have already noted a few of these debates in optics and electricity, and they played an even larger role in the development of seventeenth-century chemistry and of early nineteenth-century geology.3 Furthermore, debates like these do not vanish once and for all with the appearance of a paradigm. Though almost non-existent during periods of normal science, they recur regularly just before and during scientific revolutions, the periods when paradigms are first under attack and then subject to change. The transition from Newtonian to quantum mechanics evoked many debates about both the nature and the standards of physics, some of which still continue.4 There are people alive today who can remember the similar arguments engendered by Maxwell’s electromagnetic theory and by statistical mechanics.5 And earlier still, the assimilation of Galileo’s and Newton’s mechanics gave rise to a particularly famous series of debates with Aristotelians, Cartesians, and Leibnizians about the standards legitimate to science.6 When scientists disagree about whether the fundamental problems of their field have been solved, the search for rules gains a function that it does not ordinarily possess. While paradigms remain secure, however, they can function without agreement over rationalization or without any attempted rationalization at all.
A fourth reason for granting paradigms a status prior to that of shared rules and assumptions can conclude this section. The introduction to this essay suggested that there can be small revolutions as well as large ones, that some revolutions affect only the members of a professional subspecialty, and that for such groups even the discovery of a new and unexpected phenomenon may be revolutionary. The next section will introduce selected revolutions of that sort, and it is still far from clear how they can exist. If normal science is so rigid and if scientific communities are so close-knit as the preceding discussion has implied, how can a change of paradigm ever affect only a small subgroup? What has been said so far may have seemed to imply that normal science is a single monolithic and unified enterprise that must stand or fall with any one of its paradigms as well as with all of them together. But science is obviously seldom or never like that. Often, viewing all fields together, it seems instead a rather ramshackle structure with little coherence among its various parts. Nothing said to this point should, however, conflict with that very familiar observation.
On the contrary, substituting paradigms for rules should make the diversity of scientific fields and specialties easier to understand. Explicit rules, when they exist, are usually common to a very broad scientific group, but paradigms need not be. The practitioners of widely separated fields, say astronomy and taxonomic botany, are educated by exposure to quite different achievements described in very different books. And even men who, being in the same or in closely related fields, begin by studying many of the same books and achievements may acquire rather different paradigms in the course of professional specialization. Consider, for a single example, the quite large and diverse community constituted by all physical scientists. Each member of that group today is taught the laws of, say, quantum mechanics, and most of them employ these laws at some point in their research or teaching. But they do not all learn the same applications of these laws, and they are not therefore all affected in the same ways by changes in quantum-mechanical practice. On the road to professional specialization, a few physical scientists encounter only the basic principles of quantum mechanics. Others study in detail the paradigm applications of these principles to chemistry, still others to the physics of the solid state, and so on. What quantum mechanics means to each of them depends upon what courses he has had, what texts he has read, and which journals he studies. It follows that, though a change in quantum-mechanical law will be revolutionary for all of these groups, a change that reflects only on one or another of the paradigm applications of quantum mechanics need be revolutionary only for the members of a particular professional subspecialty. For the rest of the profession and for those who practice other physical sciences, that change need not be revolutionary at all. In short, though quantum mechanics (or Newtonian dynamics, or electromagnetic theory) is a paradigm for many scientific groups, it is not the same paradigm for them all. Therefore, it can simultaneously determine several traditions of normal science that overlap without being coextensive. A revolution produced within one of these traditions will not necessarily extend to the others as well. One brief illustration of specialization’s effect may give this whole series of points additional force. An investigator who hoped to learn something about what scientists took the atomic theory to be asked a distinguished physicist and an eminent chemist whether a single atom of helium was or was not a molecule. Both answered without hesitation, but their answers were not the same. For the chemist the atom of helium was a molecule because it behaved like one with respect to the kinetic theory of gases. For the physicist, on the other hand, the helium atom was not a molecule because it displayed no molecular spectrum.7 Presumably both men were talking of the same particle, but they were viewing it through their own research training and practice. Their experience in problem-solving told them what a molecule must be. Undoubtedly their experiences had had much in common, but they did not, in this case, tell the two specialists the same thing. As we proceed we shall discover how consequential paradigm differences of this sort can occasionally be.
Notes
2 Ludwig Wittgenstein, Philosophical Investigations, trans. G. E. M. Anscombe (New York, 1953), pp. 31–36. Wittgenstein, however, says almost nothing about the sort of world necessary to support the naming procedure he outlines. Part of the point that follows cannot therefore be attributed to him.
3 For chemistry, see H. Metzger, Les doctrines chimiques en France du début du XVII e à la fin du XVIII e siècle (Paris, 1923), pp. 24–27, 146–49; and Marie Boas, Robert Boyle and Seventeenth-Century Chemistry (Cambridge, 1958), chap. ii. For geology, see Walter F. Cannon, “The Uniformitarian-Catastrophist Debate,” Isis 51 (1960), 38–55; and C. C. Gillispie, Genesis and Geology (Cambridge, Mass., 1951), chaps, iv–v.
4 For controversies over quantum mechanics, see Jean Ullmo, La crise de la physique quantique (Paris, 1950), chap. II.
5 For statistical mechanics, see René Dugas, La théorie physique au sens de Boltzmann et ses prolongements modernes (Neuchatel, 1959), pp. 158–84, 206–19. For the reception of Maxwell’s work, see Max Planck, “Maxwell’s Influence in Germany,” in James Clerk Maxwell: A Commemoration Volume, 1831–1931 (Cambridge, 1931), pp. 45–65, esp. pp. 58– 63; and Silvanus P. Thompson, The Life of William Thomson Baron Kelvin of Largs (London, 1910), II, 1021–27.
6 For a sample of the battle with the Aristotelians, see A. Koyré, “A Documentary History of the Problem of Fall from Kepler to Newton,” Transactions of the American Philosophical Society, XLV (1955), 329–95. For the debates with the Cartesians and Leibnizians, see Pierre Brunet, L’introduction des théories de Newton en France au XVII e siècle (Paris, 1931); and A. Koyré, From the Closed World to the Infinite Universe (Baltimore, 1957), chap. XI.
7 The investigator was James K. Senior, to whom I am indebted for a verbal report. Some related issues are treated in his paper, “The Vernacular of the Laboratory,” Philosophy of Science, XXV (1958), 163–68.